Arctic winter high-latitude seasonal stratospheric aerosol injection
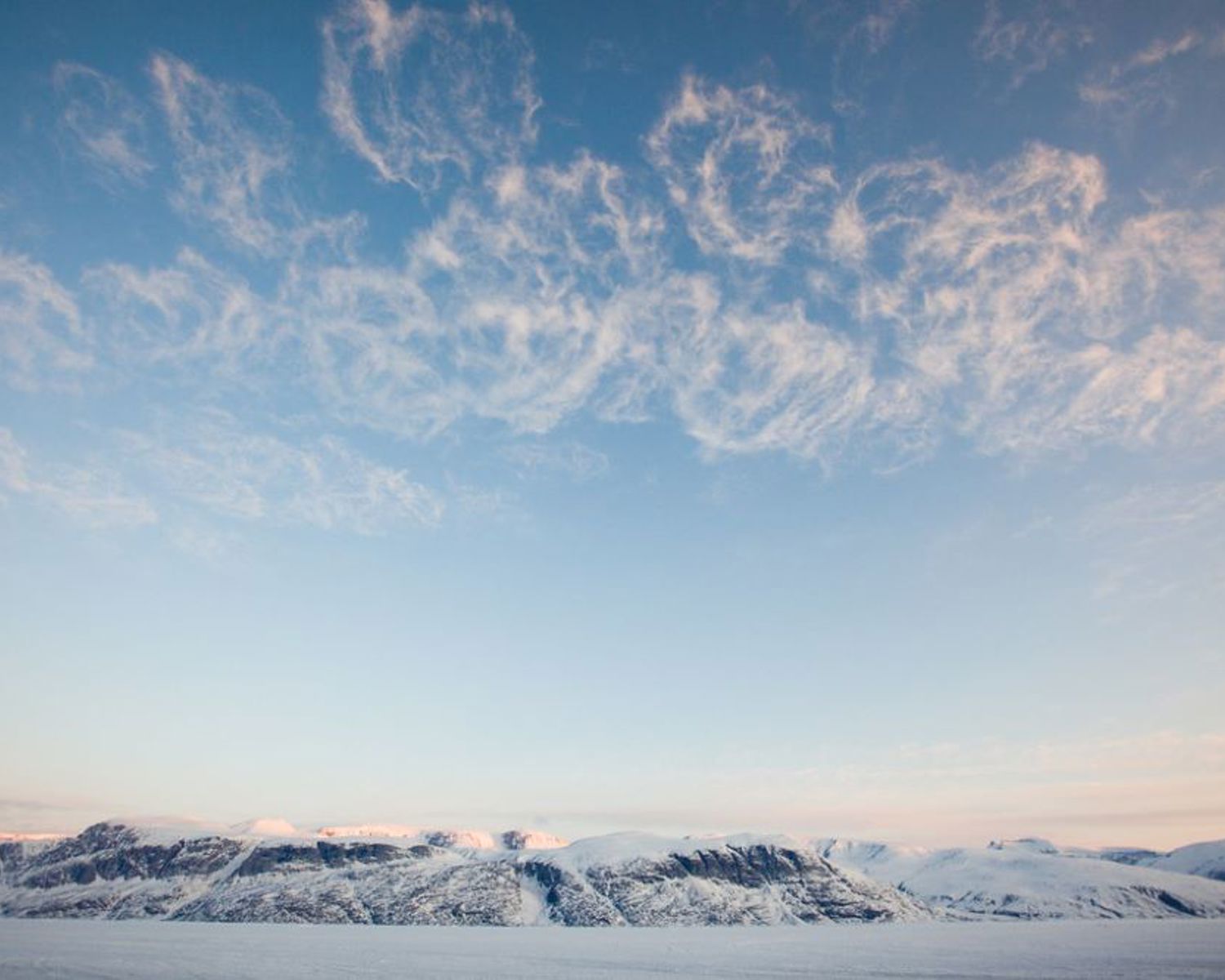
Uummannaq is home to 1400 people and 3000 dogs. This district boasts the largest number of glaciers found in the one place.
Year: 2011
Photographer: Lawrence Hislop
GHG emissions reductions and future negative emissions are the only sustainable solutions to stabilize or even reverse global warming as they counter the cause of the problem. However, the required actions will likely take time to materialize, and inertia in the climate system and locked-in warming already ensures major changes in global temperatures and the nearing of several tipping points. Solar radiation management (SRM) techniques seek to reduce global temperatures by reflecting incoming solar radiation. They would thereby not fix the underlying issue of the warming effects of GHGs but are, according to the United Nations Environment Programme (UNEP) Review on Solar Radiation Modification Research (2023), 'the only known approach that could be used to cool the Earth within a few years'.
Stratospheric aerosol injection (SAI) is an idea to inject particles in the stratosphere to reduce the amount of incoming solar energy (Rasch et al. 2008; Irvine et al. 2016). The principle behind SAI is well understood as it is analogous to some naturally occurring volcanic eruptions which have been consistently observed to cool global temperatures over year-timescales if they deposit material high enough into the atmosphere (Robock et al. 2013; IPCC AR6 WG1, chapter 4). Although multiple aerosol types have been suggested for SAI, the most studied idea would burn or inject sulfur into the stratosphere where it would form SO2 which would then oxidize into sulphuric acid and form a relatively stable layer. Due to the high seasonality in the higher latitude regions, specific strategies look at injection rates that see greatest injections in spring and summer, and no injections before and during winter (Lee et al. 2021).
Analysis overview

Technological Readiness Level (TRL)
Low 1
At the moment, SAI studies are mostly limited to model simulations. There have recently been several controversial small-scale initiatives that have prompted major debate within the community (See the coverage of the launches of Make Sunsets in the US and Mexico and the SATAN project launch in the UK), but so far no major or substantial outdoor tests have been conducted (Low et al, 2022). Model studies allow scholars to get an increasingly accurate understanding of the effect of SAI on the climate system and a formulation of potential injection scenarios. These models have become more sophisticated, and are also jointly investigated in the Geoengineering Model Intercomparison Project (GeoMIP) and the Geoengineering Large Ensemble Simulations (GLENS). However, many topics like aerosol behavior and aerosol–cloud–radiation interaction are still poorly understood (IPCC AR6 WG3, chapter 14), and would be hard to study without outdoor experiments. There have been several suggestions with regards to injection devices, with specific aircraft generally considered as most probable for altitudes up to 20 km (Smith and Wagner 2018). Although higher injections would be more effective according to model studies, Smith et al. (2022) warn 'raising the deployment altitude from 20 to 25 km entails a step change in both costs and safety hazards'.
Deployment seems far off, and although Smith et al. (2022) call a high latitude injection program 'logistically feasible', they envision a high latitude injection program to be a 'decadal time-scale project'. Opinions on if and how to pursue further research vary widely, with a call for a moratorium on further research being broadly signed in 2022 (https://www.solargeoeng.org/), whilst on the other side calls intensify for further research, recently most notably in the form of a UNEP (2023) report and in an open letter signed by, amongst others, James Hansen (https://climate-intervention-research-letter.org/). Such research projects could either be national or international, with a report by the US National Academies of Sciences, Engineering, and Medicine for example suggesting that 'the U.S. should pursue a research program for solar geoengineering — in coordination with other nations' (NASEM, 2021). There seems to be an increasing attention to the inclusion of different actors in a previously global North dominated field, as exemplified by the Degrees Initiative (https://www.degrees.ngo/) that seeks to encourage the evaluation of SAI in “developing countries”.
Technological Readiness Level (TRL)
A technology with a TRL of 1-3: TRL 1 – Basic; TRL 2 – Concept formulated; TRL 3 – Experimental proof of concept

Scalability
Medium 2
The scalability of SAI is limited in one sense as the effectiveness of injections decreases with increased injection rates (Kleinschmitt et al., 2018). These effects are however most pronounced at high injection rates that would be required for the complete mitigation of high forcing scenarios.
The distribution of aerosols in the stratosphere at low latitudes would ensure a near-global coverage and a global reduction of surface temperatures. If aerosols were to be specifically injected at higher latitudes, they would have more focussed effects over those parts, as stratospheric dynamics move the particles pole-wards. Although estimates vary, it is estimated that a relatively manageable fleet of hundreds of aircraft would be needed to achieve significant surface cooling (Smith and Wagner, 2018).
Scalability
Physically somewhat scalable; linear efficiency

Timeliness for near-future effects
High 3
Although there are currently no feasible injection measures for SAI, the development or modification of existing aircraft to release aerosols in the stratosphere is likely to be a relatively straightforward task (Smith et al., 202), and Smith et al. (2022) consider a high latitude injection program to be a 'decadal time-scale project'. A lot would depend on the proposed SAI injection strategy and goal. UNEP (2023) distinguishes broadly between two framings of SRM deployment, either as an emergency measure, or as a durable part of global climate action strategies to reduce the worst of the warming effects and provide more time for emissions reductions and negative emission technologies to be employed.
Timeliness for near-future effects
Implemented in time to make a significant difference

Northern + Arctic potential
High 3
Model studies have consistently shown SAI could cool surface temperatures, and slow or reverse the thawing of the cryosphere in the Northern and Arctic (Robock et al., 2008; Berdahl et al., 2014; Chen et al., 2020; Lee et al., 2023; Chen et al., 2023). This cooling could furthermore have multiple other benefits for the region, as Tang et al. (2023) for example show it would reduce wildfires (see Wildfire Management), and Irvine et al. (2019) conclude that SAI could generally significantly decrease climate hazards.
Irvine et al. (2018) however note that effects of SAI are different from simply reversing GHG forcing. It is for instance not certain if and how injections may counter sea level rise and marine ice shelf instability, as Moore et al. (2010) showed that only very high injection scenarios would be able to delay sea level rise significantly. Yue et al. (2021) equally find that the Icelandic Vatnajökull ice cap's melt can be reduced somewhat by SAI, although it remains relatively insensitive to solar geoengineering. Concerning the wider cryosphere, Zhao et al. (2017) find SRM could also slow melt in other areas like in the “Third Pole” region.
Northern + Arctic potential
Very detectable impacts in the Arctic, above the global average; technology ideally/preferably located here

Global potential
High 3
Multiple model studies and historical analogs of volcanic eruptions show SAI could swiftly reduce global temperatures when initiated (IPCC AR6 WG3, chapter 14). The amount of cooling would be dependent on injection strategies and on the total amount of sulfur injected, with larger injection amounts achieving a progressively lower efficacy (Kleinschmitt et al., 2018). The IPCC AR6 WG1 (2021) report therefore attains they have high confidence that SRM technologies like SAI could offset some of the effects of GHG forcing, and gives maximum forcing potentials ranging from –5 to –2 W m–2 (Chapter 6), and a global mean radiative forcing potential of 1–8 W m–2 (Chapter 4).
Global potential
Major impacts detected

Cost - benefit
Low 3
Cost - benefit
Low investment cost compared to the avoided damage cost (e.g., a few %) and/or inexpensive relative to other measures with similar impact

Environmental risks
Medium 2
Some of the major objections against SAI relate to this measure’s possible environmental and ecological effects. The expected ones are still relatively under-researched (Zarnetske et al., 2020), and there might be several unknown risks related to global deployment.
A first expected consequence of SAI would be an effect on rainfall patterns and a weakening of monsoons (Bala et al., 2008; Krishnamohan and Bala, 2020; Riky, 2023). Such disturbance would be especially strong in case of uneven global cooling, and could be lessened by specific injection strategies (IPCC, AR6 WG1, 2021 Ch. 4). Nalam et al. (2018) for instance found that SAI geoengineering in the Arctic alone would significantly alter precipitation patterns around the globe, but that this could be largely balanced out by a mirroring cooling of the Antarctic.
Although there have been fears of acid rain due to the use of sulfur, already the earliest estimates on SAI by Budyko (1974) indicated that the total amounts of sulfur used are negligible in comparison to other anthropogenic emissions, and a recent study indicated that even in the most ambitious emission pathways, global sulfur depositions would not vary much from present levels (Visioni, 2020).
The use of sulfur as aerosol could also impact stratospheric ozone levels (Tilmet et al., 2008). The IPCC AR6 WG1 report (chapter 4) says its “likely” SAI would delay the recovery of the Antarctic ozone hole, and model studies find that this effect could be particularly pronounced in the first decade, and delay recovery by several more (Tilmes et al. 2021; Times et al. 2022).
Sustained deployment could have significant impact on the lower and middle stratosphere, and UNEP (2023) writes this could have 'unknown consequences for the environment on and near Earth’s surface.'
Since SAI would reduce the amount of available energy for photosynthesis on the Earth’s surface and in the oceans, the IPCC 2021 AR6 WG1 report states it has medium confidence that SAI would ‘cause a reduction in plant and soil respiration and slow the reduction of ocean carbon uptake due to warming.’
Environmental risks
More widespread and possibly regional impacts that extend beyond the immediate solution deployment location

Community impacts
Unknown 0
There is a lack of research on the potential effects of SAI on local communities. Of the many understudied topics, recently, the potential impact of SAI on human health has started to be explored (Tracy et al., 2022), with Carlson et al. (2022) for example finding it could have a significant impact on malaria distributions.
More research has been done on perceptions of geoengineering, with some studies on the relation of indigenous peoples to geoengineering (Whyte 2012; Whyte (2018), and on the perception of geoengineering by Northern communities (Buck, 2018; Mettiäinen, 2022). Certain events also reveal that there is significant skepticism against SAI amongst some communities. This showed especially clearly in the 2021 protest against the Harvard SCoPEx group in North Sweden by Sami and environmental activists. And statements like Smith et al.’s (2022) that ‘an SAI program with global benefits that would entail deployment directly overhead of far less than 1% of the world's population and nearly none of its agriculture may prove an easier sell to a skeptical world than a full-on global deployment’, further highlight the need for further research into the opinions and possible effects of potential SAI deployment in the Arctic and Northern regions.

Ease of reversibility
Easy 3
Ease of reversibility
Easily reversible naturally

Risk of termination shock
High 1
A major issue with SAI is the potential of a termination shock when the technology is abruptly halted for whatever reason (Jones et al., 2013; IPCC AR6, WG1, chapter 4; UNEP, 2023). The C2G2 risk analysis report on SRM (2022) states clearly that 'A sudden and sustained termination of large amount of SAI or MCB under a high GHG emission background would cause a rapid increase in temperature and precipitation at a rate that far exceeds that predicted for future climate change without SRM.' For the Arctic specifically, Berdahl et al. (2014) show that in a RCP4.5 scenario, a sudden termination would negate all geoengineered benefits in terms of retained snow and ice within a decade.
Risk of termination shock
High or very significant termination shock or damage

Legality/governance
Low 1
A main objection many opponents of SAI have against the technology is that it would be ungovernable, as countries and actors would dispute over target temperatures, and struggle to find common ground with regards to compensation of negative effects of SAI (Biermann et al., 2022). Others, including NASEM (2021) and UNEP (2023), instead argue for further research into the governance of geoengineering and geoengineering research. There are already several governance proposals (Reynolds, 2019) and further research is being conducted, perhaps most prominently by the Carnegie Climate Geoengineering Governance Initiative (C2G2, (https://www.c2g2.net/). UNEP (2023) however urges increasing emphasis on the need to include previously excluded voices, especially in the global South, and that decision be made ‘in a globally inclusive, equitable and transparent manner’.
Legality/governance
Illegal or banned, or the legal regime is not suited to deployment

Scientific/media attention
High 3
SAI is the most researched and written about climate intervention/geoengineering measure. Apart from several rogue field trials, there are increasing numbers of scholars around the world doing modelling work on SAI, and studying the governance issues around research and deployment.
Scientific/media attention
Numerous scientific papers with substantial funding and ongoing research groups; significant media attention and "hype"; many companies exploring commercialization options
References
Bala, G., Duffy, P. B., & Taylor, K. E. (2008). Impact of geoengineering schemes on the global hydrological cycle. Proceedings of the National Academy of Sciences, 105(22), 7664-7669. https://doi.org/10.1073/pnas.0711648105
Biermann, Frank, et al. "Solar geoengineering: The case for an international non‐use agreement." Wiley Interdisciplinary Reviews: Climate Change 13.3 (2022): e754. https://doi.org/10.1002/wcc.754
Berdahl, M., Robock, A., Ji, D., Moore, J. C., Jones, A., Kravitz, B., & Watanabe, S. (2014). Arctic cryosphere response in the Geoengineering Model Intercomparison Project G3 and G4 scenarios. Journal of Geophysical Research: Atmospheres, 119(3), 1308-1321. https://doi.org/10.1002/2013JD020627
Buck, H. J. (2018). Perspectives on solar geoengineering from Finnish Lapland: Local insights on the global imaginary of Arctic geoengineering. Geoforum, 91, 78-86. https://doi.org/10.1016/j.geoforum.2018.02.020
Carlson, C. J., Colwell, R., Hossain, M. S., Rahman, M. M., Robock, A., Ryan, S. J., ... & Trisos, C. H. (2022). Solar geoengineering could redistribute malaria risk in developing countries. Nature communications, 13(1), 2150. https://doi.org/10.1038/s41467-022-29613-w
Chen, Y., Ji, D., Zhang, Q., Moore, J. C., Boucher, O., Jones, A., ... & Tilmes, S. (2023). Northern-high-latitude permafrost and terrestrial carbon response to two solar geoengineering scenarios. Earth System Dynamics, 14(1), 55-79. https://doi.org/10.5194/esd-14-55-2023
Chen, Y., Liu, A., & Moore, J. C. (2020). Mitigation of Arctic permafrost carbon loss through stratospheric aerosol geoengineering. Nature Communications, 11(1), 2430.nterdisciplinary Reviews: Climate Change 13.3 (2022): e754. https://doi.org/10.1038/s41467-020-16357-8
Felgenhauer, T., Bala, G., Borsuk, M., Brune, M., Camilloni, I., Wiener, J.B., Xu, J. (2022). Solar Radiation Modification: A Risk-Risk Analysis, Carnegie Climate Governance Initiative (C2G), March, New York, NY: www.c2g2.net
IPCC, 2021: Climate Change 2021: The Physical Science Basis. Contribution of Working Group I to the Sixth Assessment Report of the Intergovernmental Panel on Climate Change [Masson-Delmotte, V., P. Zhai, A. Pirani, S.L. Connors, C. Péan, S. Berger, N. Caud, Y. Chen, L. Goldfarb, M.I. Gomis, M. Huang, K. Leitzell, E. Lonnoy, J.B.R. Matthews, T.K. Maycock, T. Waterfield, O. Yelekçi, R. Yu, and B. Zhou (eds.)]. Cambridge University Press, Cambridge, United Kingdom and New York, NY, USA, 2391 pp. https://doi.org/10.1017/9781009157896
Irvine, P., Emanuel, K., He, J., Horowitz, L. W., Vecchi, G., & Keith, D. (2019). Halving warming with idealized solar geoengineering moderates key climate hazards. Nature Climate Change, 9(4), 295-299. https://doi.org/10.1038/s41558-019-0398-8.
Irvine, P. J., Kravitz, B., Lawrence, M. G., & Muri, H. (2016). An overview of the Earth system science of solar geoengineering. Wiley Interdisciplinary Reviews: Climate Change, 7(6), 815-833. https://doi.org/10.1002/wcc.423
Jones, A., Haywood, J. M., Alterskjær, K., Boucher, O., Cole, J. N., Curry, C. L., ... & Yoon, J. H. (2013). The impact of abrupt suspension of solar radiation management (termination effect) in experiment G2 of the Geoengineering Model Intercomparison Project (GeoMIP). Journal of Geophysical Research: Atmospheres, 118(17), 9743-9752. https://doi.org/10.1002/jgrd.50762
Kleinschmitt, C., Boucher, O., & Platt, U. (2018). Sensitivity of the radiative forcing by stratospheric sulfur geoengineering to the amount and strategy of the SO 2 injection studied with the LMDZ-S3A model. Atmospheric Chemistry and Physics, 18(4), 2769-2786. https://doi.org/10.5194/acp-18-2769-2018
Krishnamohan, K. S., & Bala, G. (2022). Sensitivity of tropical monsoon precipitation to the latitude of stratospheric aerosol injections. Climate Dynamics, 59(1-2), 151-168. https://doi.org/10.1007/s00382-021-06121-z
Low, S., Baum, C. M., & Sovacool, B. K. (2022). Taking it outside: exploring social opposition to 21 early-stage experiments in radical climate interventions. Energy Research & Social Science, 90, 102594. https://doi.org/10.1016/j.erss.2022.102594
Mettiäinen, I., Buck, H. J., MacMartin, D. G., & Ricke, K. L. (2022). ‘Bog here, marshland there’: tensions in co-producing scientific knowledge on solar geoengineering in the Arctic. Environmental research letters, 17(4), 045001. https://doi.org/10.1088/1748-9326/ac5715
Moore, J. C., Jevrejeva, S., & Grinsted, A. (2010). Efficacy of geoengineering to limit 21st century sea-level rise. Proceedings of the National Academy of Sciences, 107(36), 15699-15703. https://doi.org/10.1073/pnas.1008153107
Nalam A, Bala G and Modak A 2018 Effects of Arctic geoengineering on precipitation in the tropical monsoon regions Clim. Dyn. 50:3375–95. https://doi.org/10.1007/s00382-017-3810-y
National Academies of Science, Engineering and Mathematics (NASEM), 2021. Reflecting sunlight: recommendations for solar geoengineering research and research governance. Washington, DC. The National Academies Press. https://doi.org/10.17226/25762.
Rasch, P. J., Tilmes, S., Turco, R. P., Robock, A., Oman, L., Chen, C. C., ... & Garcia, R. R. (2008). An overview of geoengineering of climate using stratospheric sulphate aerosols. Philosophical Transactions of the Royal Society A: Mathematical, Physical and Engineering Sciences, 366(1882), 4007-4037. https://doi.org/10.1098/rsta.2008.0131
Reynolds, J. L. (2019). The governance of solar geoengineering: managing climate change in the Anthropocene. Cambridge University Press. 250pp. ISBN 978-1-10716-195-5
Ricke, K., Wan, J. S., Saenger, M., & Lutsko, N. J. (2023). Hydrological Consequences of Solar Geoengineering. Annual Review of Earth and Planetary Sciences, 51.
Robock, A., MacMartin, D. G., Duren, R., & Christensen, M. W. (2013). Studying geoengineering with natural and anthropogenic analogs. Climatic Change, 121, 445-458.
Robock, A., Oman, L., & Stenchikov, G. L. (2008). Regional climate responses to geoengineering with tropical and Arctic SO2 injections. Journal of Geophysical Research: Atmospheres, 113(D16). https://doi.org/10.1007/s10584-013-0777-5
Tang, W., Tilmes, S., Lawrence, D. M., Li, F., He, C., Emmons, L. K., Buchholz, R. R., and Xia, L.(2023). Impact of solar geoengineering on wildfires in the 21st century in CESM2/WACCM6, Atmos. Chem. Phys., 23, 5467–5486, https://doi.org/10.5194/acp-23-5467-2023
Tilmes, S., Müller, R., & Salawitch, R. (2008). The sensitivity of polar ozone depletion to proposed geoengineering schemes. Science, 320(5880), 1201-1204. https://doi.org/10.1126/science.115396
Tilmes, S., Richter, J. H., Kravitz, B., MacMartin, D. G., Glanville, A. S., Visioni, D. et al. Sensitivity of Total Column Ozone to Stratospheric Sulfur Injection Strategies. Geophysical Research Letters. 2021; 48: 1–10. https://doi.org/10.1029/2021GL094058
Tilmes, S., Visioni, D., Jones, A., Haywood, J., Seferian, R., Nabat, P. et al. Stratospheric ozone response to sulfate aerosol and solar dimming climate interventions based on the G6 Geoengineering Model Intercomparison Project (GeoMIP) simulations. Atmospheric Chemistry and Physics. 2022; 22: 4557–4579. https://doi.org/10.5194/acp-22-4557-2022
Tracy, S. M., Moch, J. M., Eastham, S. D., & Buonocore, J. J. (2022). Stratospheric aerosol injection may impact global systems and human health outcomes. Elem Sci Anth, 10(1), 00047. https://doi.org/10.1525/elementa.2022.00047
United Nations Environment Programme (2023). One Atmosphere: An Independent Expert Review on Solar Radiation Modification Research and Deployment. https://wedocs.unep.org/20.500.11822/41903.
Visioni, D., Slessarev, E., MacMartin, D. G., Mahowald, N. M., Goodale, C. L., & Xia, L. (2020). What goes up must come down: impacts of deposition in a sulfate geoengineering scenario. Environmental Research Letters, 15(9), 094063. https://doi.org/10.1088/1748-9326/ab94eb
Whyte, K. P. (2018). Indigeneity in geoengineering discourses: Some considerations. Ethics, Policy & Environment, 21(3), 289-307. https://doi.org/10.1080/21550085.2018.1562529
Whyte, K. (2012). Indigenous peoples, solar radiation management, and consent. Engineering the Climate: The Ethics of Solar Radiation Management, Lexington Books.
Yue, C., Schmidt, L. S., Zhao, L., Wolovick, M., & Moore, J. C. (2021). Vatnajökull mass loss under solar geoengineering due to the North Atlantic meridional overturning circulation. Earth's Future, 9(9), e2021EF002052. https://doi.org/10.1029/2021EF002052
Zhao, L., Yang, Y., Cheng, W., Ji, D., and Moore, J. C.: Glacier evolution in high-mountain Asia under stratospheric sulfate aerosol injection geoengineering, Atmos. Chem. Phys., 17, 6547–6564, https://doi.org/10.5194/acp-17-6547-2017