Black carbon reduction
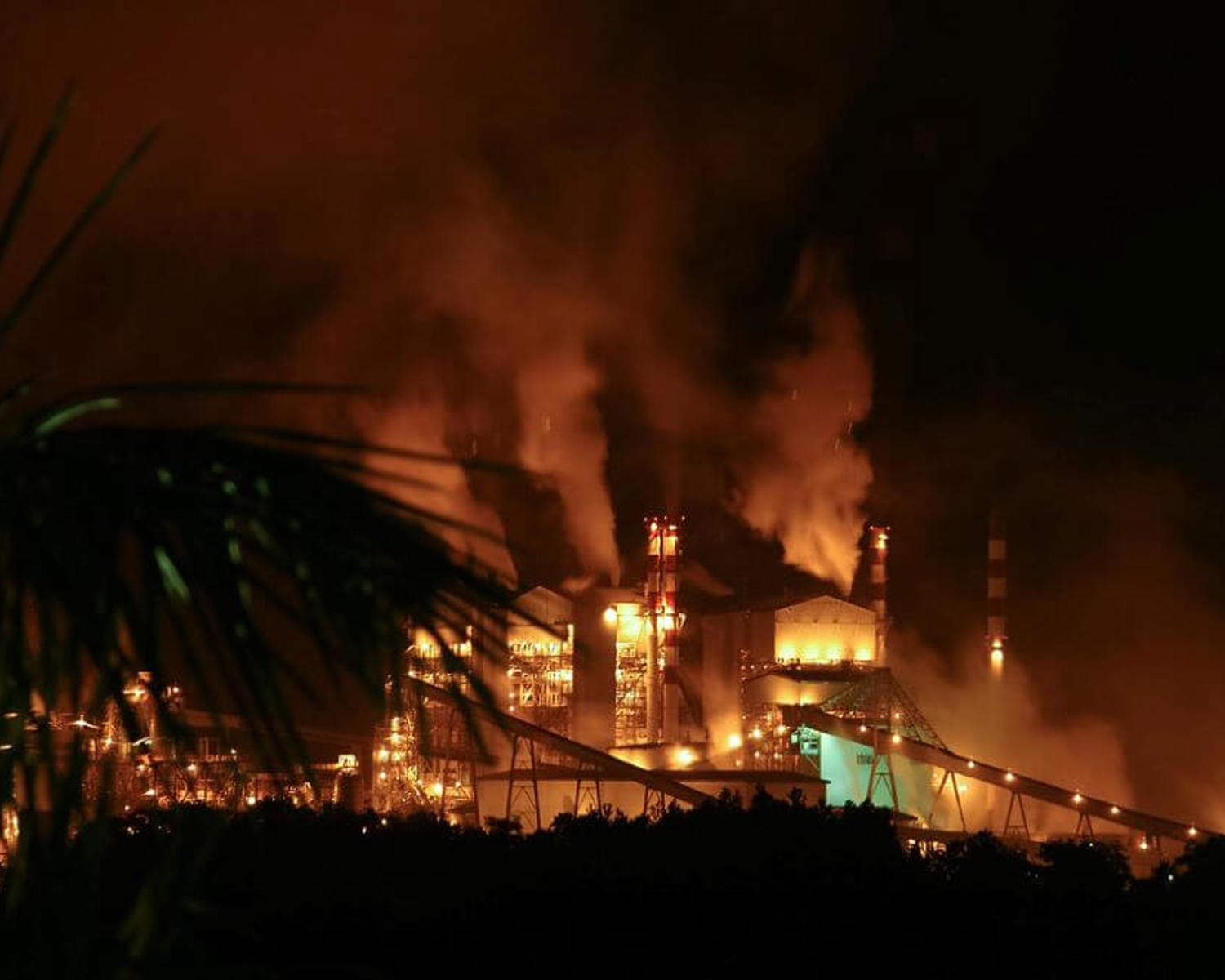
The forests on the Indonesian island of Sumatra are witnessing some of the fastest rates of deforestation in the world. Over the last decade, dozens of pulp and paper companies have established themselves on the island chopping down trees and setting up palm oil plantations on the cleared land. These changes have far-reaching environmental as well as socio-economic impacts on the island communities that are so dependent on the natural resources and ecosystem services provided by the rainforests.
Year: 2014
Photographer: Peter Prokosch
Black Carbon (BC), also known as soot, is produced through the incomplete combustion of fossil fuels and biomaterials. Apart from its negative health impacts, BC also has significant climate effects because it generally has a lower albedo than its surroundings, which increases the amount of radiation absorbed both when BC is present in the atmosphere and when it is deposited on land (Stjern et al. 2017). Due to the large albedo differences, the effects of BC are especially significant in areas that are normally covered in snow or ice (Hadley and Kirchstetter 2012; Sand et al. 2016; Kang et al. 2020).
The importance of reducing BC emissions from natural sources like forest fires is discussed elsewhere (see Forest Fire Management). BC emission reductions from anthropogenic sources can be achieved in multiple ways. In high mountain regions, much attention is given to technological improvements, such as replacing biofuel systems with cleaner-burning alternatives. In the Arctic, legal and governance approaches, such as banning certain fuels in shipping, have been important (Messner, 2020).
Which measure would be most effective is highly dependent on the region. Makarovaа et al. (2021), for example, found that road transport was the most important anthropogenic BC source in Murmansk and that a combination of measures could be used to reduce soot emissions by 65.5%. Stohl et al. (2013) found that one of the main sources of BC in the Arctic is flaring, while Virkanrt et al. (2021) note that BC emission in Greenland come from multiple sources and is increasing due to tourism, forest fires, and ship traffic.
Analysis overview

Technological Readiness Level (TRL)
High 3
Given the large scope of possible mitigation strategies, there is no clear answer for this, although most techniques are already readily available. There is a need for increased BC emission data and observation to design adequate policies. Kang et al., (2020), for instance, note large discrepancies between studies on BC concentrations, depending on measurement methods and models used. Moreover, it is not clear how effective BC mitigation would be as a measure to cool global and Arctic temperatures due to the many indirect effects of such mitigation (Kühn et al., 2020), and whether mitigation strategies could result in a net heating effect. For example, reducing sulfate particle emissions, which until have now had a cooling effect on temperatures, may have the opposite effect (Takemure and Suzuki, 2019; von Salzen et al., 2022).
Technological Readiness Level (TRL)
A technology with a TRL of 7-9: TRL 7 – prototype demonstrated; TRL 8 – system complete; TRL 9 – system proven

Scalability
Medium 2
The difficulty of scalability depends on the strategy and the source of BC. Although BC mitigation in the Arctic is shown to have significant potential (Aakre et al., 2017; Kühn et al., 2020), most BC in the region is from sources outside of the region (Browse et al., 2013; Khan and Kulovesi, 2018), necessitating international collaboration. Rypdal et al. (2009) argue that the most effective BC mitigation strategies would focus on Asia, as reductions in this region are relatively cheaper to acheive, and because a large portion of global BC emissions originate from Asia.
Scalability
Physically somewhat scalable; linear efficiency

Timeliness for near-future effects
High 3
Some of the technologies need to be developed further, but most mitigation strategies would be relatively quick to deploy, if adequate policies were implemented. Morevoer, BC mitigation strategies would be able to achieve effects on a relatively short timescale (Kühn et al., 2020).
Timeliness for near-future effects
Implemented in time to make a significant difference

Northern + Arctic potential
Medium 2
Given the increased albedo reducing effect of BC on snowy and icy surfaces, the reduction of such particles would be of prime importance for the Arctic. There are, however, still significant uncertainties when ‘quantifying the role of BC in cryospheric melting' (Kang et al. 2020). Apart from the previously mentioned net warming effects that could come with changes in anthropogenic emissions, the role of BC on ice melt could, in some contexts, be relatively insignificant compared to other albedo-reducing particles such as dust or organic matter. Kaspari et al. (2020), for example, find that at present the effect of dust far outweighs that of BC when it comes to albedo effects on the North American South Cascade Glacier.
There are already numerous actions taken by states to address carbon, including the Arctic Council's “Enhanced Black Carbon and Methane Emissions Reductions: An Arctic Council Framework for Action”. Local mitigation strategies could also play an important role in this. Makarovaа et al. (2021), for example, showed that a mix of policies could reduce BC emissions in Murmansk by 65.5%. Aakre et al. (2017) write that major BC reductions in the Arctic can be made by specific groups of Arctic countries, and that Russia is of crucial importance for the Arctic region. Kühn et al. (2020) equally argue that Arctic states can significantly reduce regional BC concentrations by themselves.
Much attention in the Arctic has gone to shipping regulation, as 5 to 25% of air pollution in the region is shipping-related (Aliabadi et al. 2015). Comer et al. (2017) note that ‘[r]oughly two-thirds of the BC emissions (e.g.,193 tons) made in 2015 over the Arctic could be attributed to ships.’ Browse et al. (2013), however, highlight that shipping regulations in isolation might only be effective locally, such as in Greenland, where 10 to 15% of BC is attributable to shipping. They emphasize that major reductions would have to come through international regulations, as most of the BC in the Arctic is emitted from sources outside of the region. This international focus is also advocated by Khan and Kulovesi (2018), who also argue for ‘global engagement’.
Northern + Arctic potential
Statistically detectable impacts in the Arctic above the global average; no difference between deploying the solution here or elsewhere

Global potential
Low 1
According to a literature review by Kang et al. (2020), the globally averaged direct and indirect radiative forcing effects of BC are estimated to be up to 1.2 W m−2 and second only to those of CO2. However, due to the complexity of the indirect temperature effects of BC, such as cloud formation, assessing the effectiveness of mitigation efforts would be complicated, especially when compared to the mitigation of other aerosols such as methane (Smith et al., 2020). The ultimate net effects of mitigation, therefore, would likely be far smaller than assumed when only direct effects were taken into consideration (Kühn et al., 2020). Mitigation of BC would likely also impact the atmospheric presence of other aerosols such as sulfate (IPCC AR6 W3), which have had a net cooling effect on the climate (Takemure and Suzuki, 2019; von Salzen et al., 2022). Furthermore, Harmsen et al. (2020) write that 'the effect of BC mitigation on global mean temperature is found to be modest at best (with a maximum short-term GMT decrease of 0.02 °C in 2030) and could even lead to warming (with a maximum increase of 0.05 °C in case of a health-focused strategy, where all aerosols are strongly reduced).'
Global potential
Insignificant to be detected at a global scale

Cost - benefit
Unknown 0
Costs and benefits depend highly on the chosen measure. Furthermore, different measures would be paid for by different groups. Legislation of shipping fuels, for example, would likely incur costs for commercial companies, while the alteration of combustion systems in public facilities would require public funds.

Environmental risks
Medium 2
BC reduction would likely also entail reduction of other pollutants (IPCC AR6 WG3). This is generally beneficial, although the removal of certain other chemical particles like sulfite might ultimately also have a net warming effect, which could be detrimental to the environment (von Salzen et al., 2022).
Environmental risks
More widespread and possibly regional impacts that extend beyond the immediate solution deployment location

Community impacts
Beneficial 3
Apart from the climate effects of BC mitigation, a reduction of black carbon particles in the air would also lead to significant health benefits (Shindell et al. 2012; Messner, 2020; IPCC AR6 WG3). Harmsen et al. (2020) even write that stringent action could potentially avoid or have avoided as many as 4 to 12 million deaths between 2015 and 2030. Kühn et al. (2020) argue that a successful BC mitigation in ‘all Arctic Council member and observer States could reduce the annual global number of premature deaths by 329,000 by the year 2030, which amounts to 9 % of the total global premature deaths due to particulate matter.'
Community impacts
Significant benefits to communities

Ease of reversibility
Easy 3
Ease of reversibility
Easily reversible naturally

Risk of termination shock
Low 3
Risk of termination shock
Low or insignificant termination shock or damage

Legality/governance
High 3
There are several attempts to include such measures into Arctic and Northern governance, especially around shipping fuel (e.g., the Arctic-Council’s adaptation in 2015 of the “Enhanced Black Carbon and Methane Emissions Reductions: An Arctic Council Framework for Action”). Kühn et al. (2020) note that they believe the probability that such reductions will be implemented is relatively high, as organizations such as the Arctic Council have already done a lot of work on it, and member states have an active interest in its success.
Legality/governance
Currently legal to deploy, with governance structures in place to facilitate it and/or financial incentives to develop it

Scientific/media attention
Medium 2
There is significant interest into BC mitigation, especially when it comes to legislation of shipping fuels and the effects of forest fires. There have also been major global strategies from UNEP, and specific Arctic-focussed projects, including the EU-funded project "Arctic Black Carbon impacting on Climate and Air Pollution (ABC-iCAP" )abc-icap.amap.no), and the Arctic Council's “Enhanced Black Carbon and Methane Emissions Reductions: An Arctic Council Framework for Action” (Ginzburg, 2018).
Scientific/media attention
Some attention within the scientific community, including published research and funding programmes; some media attention; some commercial interest
References
Aakre, S., Kallbekken, S., Van Dingenen, R. et al. (2018). Incentives for small clubs of Arctic countries to limit black carbon and methane emissions. Nature Clim Change 8, 85–90. https://doi.org/10.1038/s41558-017-0030-8
Aliabadi, A. A., Staebler, R. M., & Sharma, S. (2015). Air quality monitoring in communities of the Canadian Arctic during the high shipping season with a focus on local and marine pollution. Atmospheric Chemistry and Physics, 15(5), 2651-2673. https://doi.org/10.5194/acp-15-2651-2015
Anenberg, S. C., Schwartz, J., Shindell, D., Amann, M., Faluvegi, G., Klimont, Z., ... & Ramanathan, V. (2012). Global air quality and health co-benefits of mitigating near-term climate change through methane and black carbon emission controls. Environmental health perspectives, 120(6), 831-839. https://doi.org/10.1289/ehp.1104301
Browse, J., Carslaw, K. S., Schmidt, A., & Corbett, J. J. (2013). Impact of future Arctic shipping on high‐latitude black carbon deposition. Geophysical research letters, 40(16), 4459-4463.https://doi.org/10.1002/grl.50876
Comer, B., Olmer, N., Mao, X., Roy, B., & Rutherford, D. (2017). Prevalence of heavy fuel oil and black carbon in Arctic shipping, 2015 to 2025. https://theicct.org/sites/default/files/publications/HFO-Arctic_ICCT_Report_01052017_vF.pdf
Ginzburg, Veronika (2018). Black Carbon and Methane. Arctic Council. https://arctic-council.org/about/task-expert/egbcm/
Hadley, O. L., & Kirchstetter, T. W. (2012). Black-carbon reduction of snow albedo. Nature Climate Change, 2(6), 437-440.https://doi.org/10.1038/nclimate1433
Harmsen, M.J.H.M., van Dorst, P., van Vuuren, D.P. et al. Co-benefits of black carbon mitigation for climate and air quality. Climatic Change 163, 1519–1538 (2020). https://doi.org/10.1007/s10584-020-02800-8
Kang, S., Zhang, Y., Qian, Y., & Wang, H. (2020). A review of black carbon in snow and ice and its impact on the cryosphere. Earth-science reviews, 210, 103346. https://doi.org/10.1016/j.earscirev.2020.103346
Kaspari, S. D., Pittenger, D., Jenk, T. M., Morgenstern, U., Schwikowski, M., Buenning, N., & Stott, L. (2020). Twentieth century black carbon and dust deposition on South Cascade Glacier, Washington State, USA, as reconstructed from a 158‐m‐long ice core. Journal of Geophysical Research: Atmospheres, 125(11), e2019JD031126. https://doi.org/10.1029/2019JD031126
Kühn, T., Kupiainen, K., Miinalainen, T., Kokkola, H., Paunu, V. V., Laakso, A., ... & Lehtinen, K. E. (2020). Effects of black carbon mitigation on Arctic climate. Atmospheric Chemistry and Physics, 20(9), 5527-5546. https://doi.org/10.5194/acp-20-5527-2020
Makarova, I., Mavrin, V., Magdin, K., & Barinov, A. (2021). Reducing black carbon emissions in the arctic territories. Transportation Research Procedia, 57, 356-362. https://doi.org/10.1016/j.trpro.2021.09.061
Messner, S. (2020). Future Arctic shipping, black carbon emissions, and climate change. In Maritime Transport and Regional Sustainability (pp. 195-208).Elsevier. https://doi.org/10.1016/C2018-0-04694-0
Rypdal, K., Rive, N., Berntsen, T. K., Klimont, Z., Mideksa, T. K., Myhre, G., & Skeie, R. B. (2009). Costs and global impacts of black carbon abatement strategies. Tellus B: Chemical and Physical Meteorology, 61(4), 625-641.DOI: 10.1111/j.1600-0889.2009.00430.x
Sand, M. et al. Response of Arctic temperature to changes in emissions of short-lived climate forcers. Nat. Clim. Change 6, 286–289 (2016). https://doi.org/10.1038/nclimate2880
Smith, S.J., Chateau, J., Dorheim, K. et al. Impact of methane and black carbon mitigation on forcing and temperature: a multi-model scenario analysis. Climatic Change 163, 1427–1442 (2020). https://doi.org/10.1007/s10584-020-02794-3
Stjern, C. W., Samset, B. H., Myhre, G., Forster, P. M., Hodnebrog, Ø., Andrews, T., ... & Voulgarakis, A. (2017). Rapid adjustments cause weak surface temperature response to increased black carbon concentrations. Journal of Geophysical Research: Atmospheres, 122(21), 11-462. https://doi.org/10.1002/2017JD027326
Stohl, A., Klimont, Z., Eckhardt, S., Kupiainen, K., Shevchenko, V. P., Kopeikin, V. M., and Novigatsky, A. N. (2013). Black carbon in the Arctic: the underestimated role of gas flaring and residential combustion emissions, Atmos. Chem. Phys., 13, 8833–8855. https://doi.org/10.5194/acp-13-8833-2013
Takemura, T., Suzuki, K. Weak global warming mitigation by reducing black carbon emissions. Sci Rep 9, 4419 (2019). https://doi.org/10.1038/s41598-019-41181-6
Vikrant, K., Kwon, E. E., Kim, K. H., Sonne, C., Kang, M., & Shon, Z. H. (2020). Air Pollution and Its Association with the Greenland Ice Sheet Melt. Sustainability, 13(1), 65. https://doi.org/10.3390/su13010065
von Salzen, K., Whaley, C. H., Anenberg, S. C., Van Dingenen, R., Klimont, Z., Flanner, M. G., ... & Winter, B. (2022). Clean air policies are key for successfully mitigating Arctic warming. Communications Earth & Environment, 3(1), 222. https://doi.org/10.1038/s43247-022-00555-x