Direct air carbon capture and storage DACCS
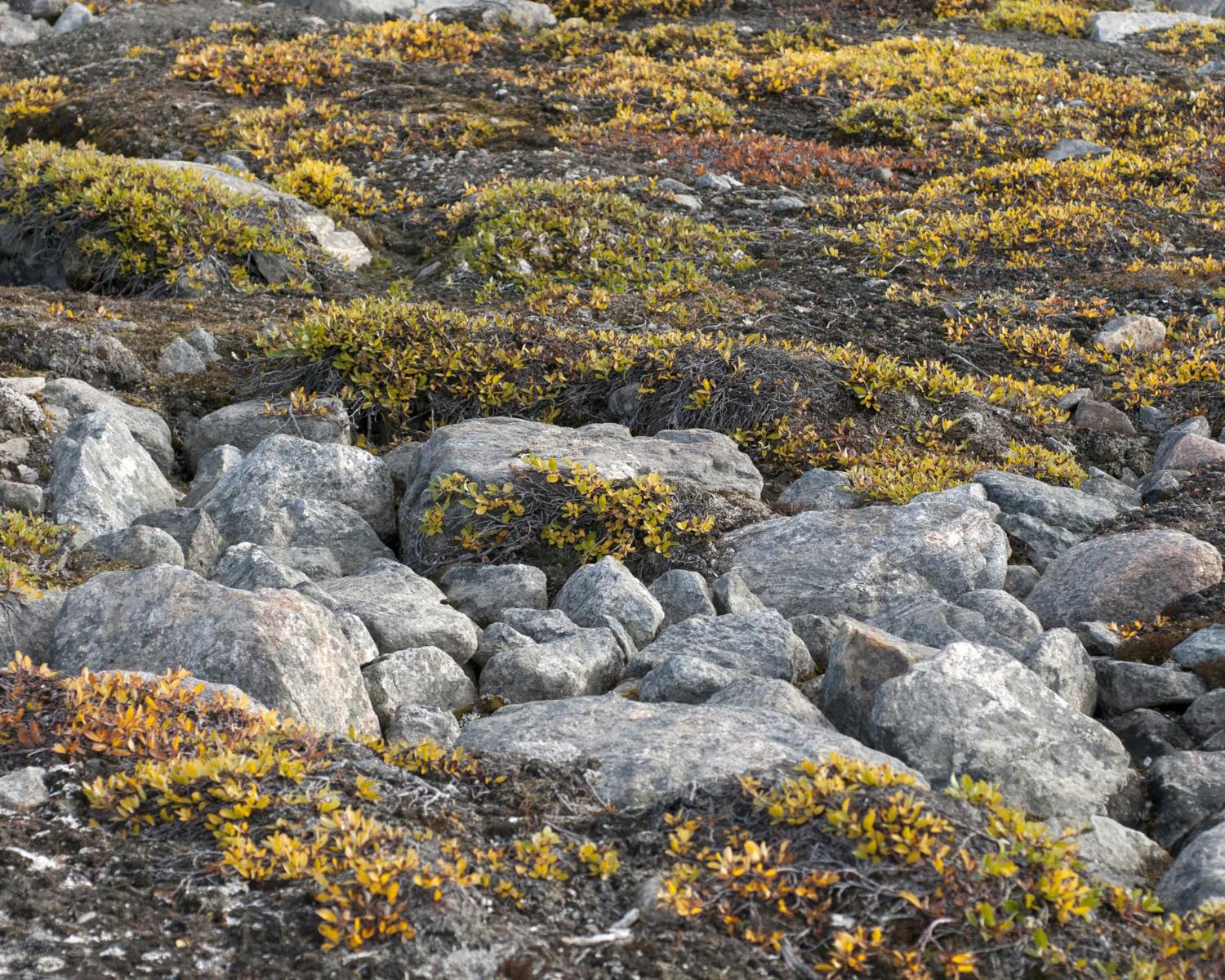
Direct air carbon capture and storage (DACCS) aims to reduce the amount of carbon dioxide in the atmosphere by taking it directly out of the air, and removing it from the carbon cycle.
Because the concentration of carbon dioxide in the air is relatively low, this involves huge ventilators that suck in large amounts of air. The carbon could then be removed from the passing air by sorbents. There are two main sorbent ideas currently being explored: absorption and adsorption, with the former dissolving the CO2 into it, and the latter adhering it to a substance. In both cases, the sorbent will release the CO2 again after energy is applied, allowing for the material to be reused. These systems therefore require significant amounts of energy, and they therefore should be coupled to renewable sources so as not to end up with net negative emissions.
The potential of cold areas like the northern and arctic regions is likely to be great for DACCS, as cold climates can provide significant benefits for the efficiency of certain technologies, and especially improve the recovery process after capturing the carbon from the air. The captured CO2 thereafter needs to be disposed of (see also Carbon capture and storage). There are several ways to do this, such as injecting it into basalt rock formations or underseas, or storing it in depleted gas fields. However, there are significant uncertainties about the large-scale feasibility of such injections, and storage underground requires monitoring to ensure no leaks occur. Alternatively, the captured carbon could be turned into resources or materials, although this raises questions about related climate effects and possible costs.
Analysis overview

Technological Readiness Level (TRL)
Medium 2
There has been a lot of hype around DACCS, and commercial companies like ClimeWorks, Carbfix, Carbon Engineering, and Global Thermostat have been featured broadly. Many issues however still need to be resolved. The State of Carbon Dioxide Removal report therefore gives it a readiness level of 6 (Smith et al. 2023).
Technological Readiness Level (TRL)
A technology with a TRL of 4-6: TRL 4 – validated in lab; TRL 5 – validated in relevant environment; TRL 6 – demonstrated in relevant environment

Scalability
Medium 2
NASEM (2019) claims that DACCS offer one of the few technologies that could potentially ' be scaled up to remove very large amounts of carbon.' And the State of Carbon Dioxide Removal report estimating potential capture of 5 to 40 GtCO2/yr (Smith et al. 2023). However, given the very limited state of current DACCS systems, there would have to be a significant scaling up of activities (Powis et al, 2023). The issue of scalability is key, as Realmonte et al (2019) show in their model study that assuming DACCS to be scalable, and finding out they aren't, would lead to an overshoot of up to 0.8°C. One requirement for scaling would be a suitable financial system that would enable investment and deployment at scale (McCormick, 2022). Another issue related to the high energy demand of DACCS. This means that scaling up could lead to energy competition (Smith et al, 2023), with Hanna et al (2021) suggesting that they could consume 14% of global electricity by 2075.///Potentially DACCS could be built everywhere (Strefler et al 2021), with the provision that they have access to renewable energy (IPCC, AR6, wg3 12.3.1.1) Specific DACCS technologies would be more efficient in certain areas like colder regions.
Scalability
Physically somewhat scalable; linear efficiency

Timeliness for near-future effects
Low 1
Timeliness for near-future effects
Implemented too late to make a significant difference

Northern + Arctic potential
High 3
Northern + Arctic potential
Very detectable impacts in the Arctic, above the global average; technology ideally/preferably located here

Global potential
High 3
Global potential
Major impacts detected

Cost - benefit
Moderate 2
Cost - benefit
Significant investment costs needed, but still much cheaper than the avoided damage costs (e.g., 30%).

Environmental risks
Low risk 3
Environmental risks
Very limited, site-specific effects restricted to the solution deployment location only

Community impacts
Neutral 2
Community impacts
Unnoticeable or negligible positive or negative effects

Ease of reversibility
Easy 3

Risk of termination shock
Low risk 3
Risk of termination shock
Low or insignificant termination shock or damage

Legality/governance
Possible 3
Legality/governance
Currently legal to deploy, with governance structures in place to facilitate it and/or financial incentives to develop it

Scientific/media attention
High 3
Scientific/media attention
Numerous scientific papers with substantial funding and ongoing research groups; significant media attention and "hype"; many companies exploring commercialization options
References
Cox, E., Spence, E., & Pidgeon, N. (2020). Public perceptions of carbon dioxide removal in the United States and the United Kingdom. Nature Climate Change, 10(8), 744-749. https://doi.org/10.1038/s41558-020-0823-z
Fuss, S.; Lamb, W.F.; Callaghan, M.W.; Hilaire, J.; Creutzig, F.; Amann, T.; Beringer, T.; De Oliveira Garcia, W.; Hartmann, J.; Khanna, T.; et al. Negative Emissions—Part 2: Costs, Potentials and Side Effects. Environ. Res. Lett. 2018, 13, 063002. https://doi.org?10.1088/1748-9326/aabf9f
Gambhir, A., & Tavoni, M. (2019). Direct air carbon capture and sequestration: how it works and how it could contribute to climate-change mitigation. One Earth, 1(4), 405-409. https://doi.org/10.1016/j.oneear.2019.11.006
Godin, J., Liu, W., Ren, S., & Xu, C. C. (2021). Advances in recovery and utilization of carbon dioxide: A brief review. Journal of Environmental Chemical Engineering, 9(4), 105644. https://doi.org/10.1016/j.jece.2021.105644
Günther, P., & Ekardt, F. (2022). Human Rights and Large-Scale Carbon Dioxide Removal: Potential Limits to BECCS and DACCS Deployment. Land, 11(12), 2153. https://doi.org/10.3390/land11122153
Hanna, R., Abdulla, A., Xu, Y., & Victor, D. G. (2021). Emergency deployment of direct air capture as a response to the climate crisis. Nature communications, 12(1), 368. https://doi.org/10.1038/s41467-020-20437-0
Kazemifar, F. (2022). A review of technologies for carbon capture, sequestration, and utilization: Cost, capacity, and technology readiness. Greenhouse Gases: Science and Technology, 12(1), 200-230. https://doi.org/10.1002/ghg.2131
Keith, D.W.; Holmes, G.; St. Angelo, D.; Heidel, K. A Process for Capturing CO2 from the Atmosphere. Joule 2018, 2, 1573–1594. https://doi.org/10.1016/j.joule.2018.05.006
McCormick C 2022 Who pays for DAC? The market and policy landscape for advancing direct air capture (National Academy of Engineering). Available at: www.nae.edu/266376/Who-Pays-for-DAC-The-Market-and-Policy-Landscape-for-Advancing-Direct-Air-Capture [Accessed 22 July 2024]
McQueen, N., Gomes, K. V., McCormick, C., Blumanthal, K., Pisciotta, M., & Wilcox, J. (2021). A review of direct air capture (DAC): scaling up commercial technologies and innovating for the future. Progress in Energy, 3(3), 032001. https://doi.org/10.1088/2516-1083/abf1ce
Möllersten, K., & Naqvi, R. (2022). Technology Readiness Assessment, Costs, and Limitations of five shortlisted NETs. Available at: https://www.researchgate.net/publication/359427009_Technology_Readiness_Assessment_Costs_and_Limitations_of_five_shortlisted_NETs_Accelerated_mineralisation_Biochar_as_soil_additive_BECCS_DACCS_Wetland_restoration [Accessed 22 July 2024].
Powis, C. M., Smith, S. M., Minx, J. C., & Gasser, T. (2023). Quantifying global carbon dioxide removal deployment. Environmental Research Letters. https://doi.org/10.1088/1748-9326/acb450
Realmonte, G., Drouet, L., Gambhir, A., Glynn, J., Hawkes, A., Köberle, A. C., & Tavoni, M. (2019). An inter-model assessment of the role of direct air capture in deep mitigation pathways. Nature communications, 10(1), 3277. https://doi.org/10.1038/s41467-019-10842-5
Scott-Buechler, Celina, Julia Jeanty, Catherine Fraser, Grace Adcox, and Charlotte Scott (2023). Advancing Equitable Deployment of Regional DAC Hubs. Data For Progress. Available at: https://www.dataforprogress.org/memos/advancing-equitable-deployment-of-regional-dac-hubs [Accessed 22 July 2024]
Smith, S. M., Geden, O., Nemet, G., Gidden, M., Lamb, W. F., Powis, C., Bellamy, R., Callaghan, M., Cowie, A., Cox, E., Fuss, S., Gasser, T., Grassi, G., Greene, J., Lück, S., Mohan, A., Müller-Hansen, F., Peters, G., Pratama, Y., Repke, T., Riahi, K., Schenuit, F., Steinhauser, J., Strefler, J., Valenzuela, J. M., and Minx, J. C. (2023). The State of Carbon Dioxide Removal - 1st Edition. The State of Carbon Dioxide Removal. https://doi.org/10.17605/OSF.IO/W3B4Z
Song, M., Rim, G., Kong, F., Priyadarshini, P., Rosu, C., Lively, R. P., & Jones, C. W. (2022). Cold-temperature capture of carbon dioxide with water coproduction from air using commercial zeolites. Industrial & Engineering Chemistry Research, 61(36), 13624-13634. https://doi.org/10.1021/acs.iecr.2c02041
Sovacool, B. K., Baum, C. M., Low, S., Roberts, C., & Steinhauser, J. (2022). Climate policy for a net-zero future: ten recommendations for Direct Air Capture. Environmental Research Letters, 17(7), 074014. https://doi.org/10.1088/1748-9326/ac77a4
Strefler, J.; Bauer, N.; Humpenöder, F.; Klein, D.; Popp, A.; Kriegler, E. Carbon Dioxide Removal Technologies Are Not Born Equal. Environ. Res. Lett. 2021, 16, 074021. https://doi.org/0.1088/1748-9326/ac0a11
Wilson, S. M. (2022). The potential of direct air capture using adsorbents in cold climates. Iscience, 25(12), 105564. https://doi.org/10.1016/j.isci.2022.105564