Ocean fertilization
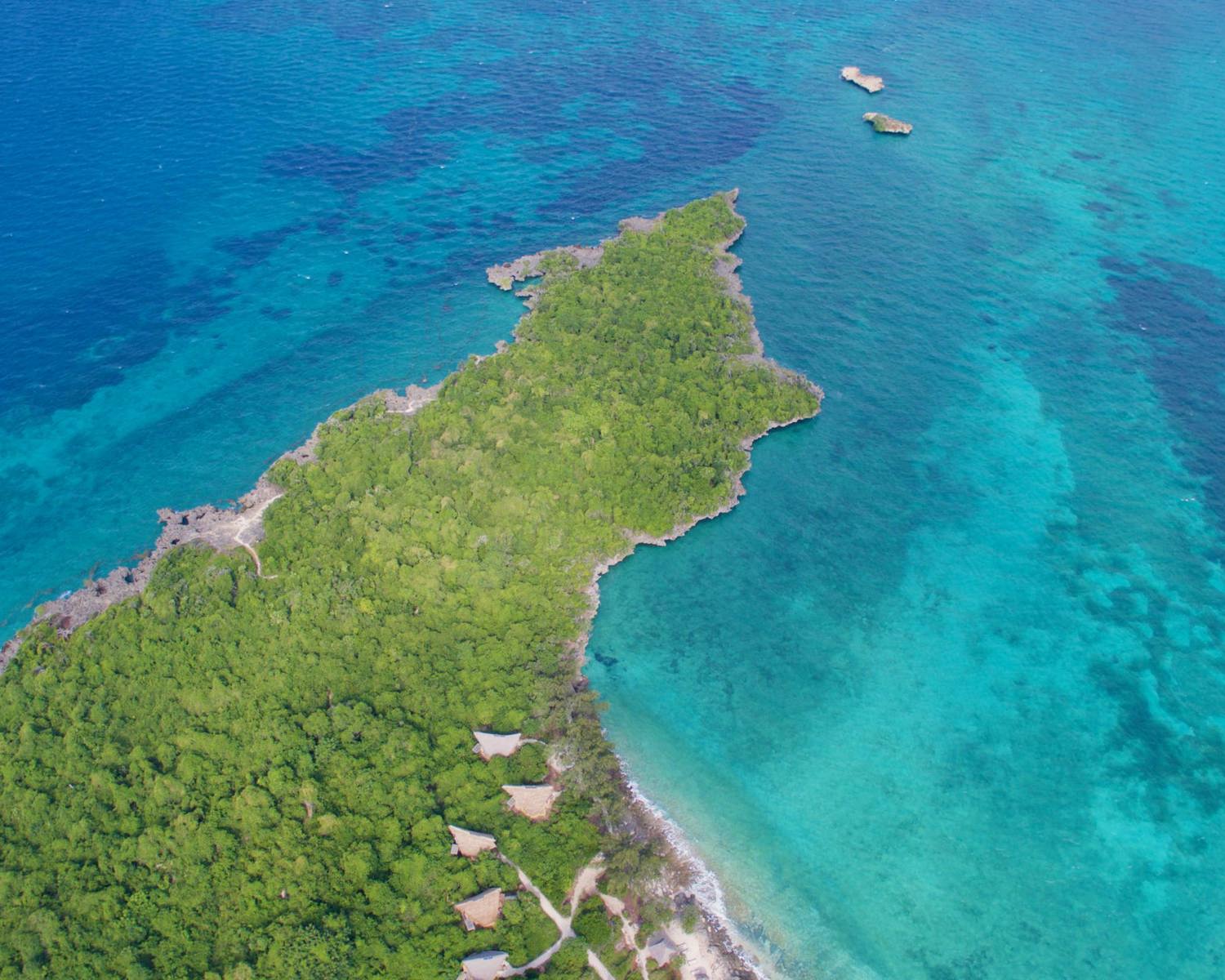
Chumbe Island Coral Park Ltd. (CHICOP) is an award-winning private nature reserve that was developed from 1991 for the conservation and sustainable management of uninhabited Chumbe Island off Zanzibar, one of the last pristine coral islands in the region.
Year: 2016
Photographer: Rob Barnes
Ocean fertilization schemes seek to increase the amount of available nutrients in the top layer of the ocean to stimulate the growth of phytoplankton.
These organisms play a major role in the oceanic carbon cycle as they utilize CO2 when photosynthesising. By encouraging greater bioproductivity, more carbon can be sequestered by organisms in the “biological pump” when they die and sink to the ocean floor and thereby remove carbon from the carbon cycle (Smetacek et al. 2012; Williamson et al. 2012). Such fertilization can also be used to stimulate higher entropic levels, for example fish production in an area (See fish management). Moreover, the UN report on marine Geoengineering speculates that further research might be done into the potential to create albedo enhancing algal blooms, which would directly reflect incoming solar radiation (GESAMP 2019; see also ocean albedo enhancement). Several different fertilization schemes have been suggested. Upwelling shall be discussed in more detail below, here the focus will be on the purposeful distribution of nutrients on the surface. Marine Phytoplankton need nitrogen (N), phosphorus (P), and iron (Fe) to grow, and there have been proposals to use all three of these elements as fertilization material. Most research has focussed on Fe fertilization, and this shall also be the focus of this section. Fe plays a crucial role in ocean biochemistry (Tagliabue et al. 2017), and occurs naturally in the atmosphere and derives from multiple sources. Ito et al. (2021) note there is still large uncertainty about such sources and its role in ocean biochemistry. Although most studies generally conceive distribution from ships, there are alternatives, like the idea to spread very fine iron-containing powder from airplanes (Emerson 2019).
Analysis overview

Technological Readiness Level (TRL)
Low 1
The technology is already proven to work locally, as natural analogues and several controversial experiments have shown that the addition of certain particles to the water can cause a localized algae bloom. However, whether this can be efficiently replicated artificially at a larger scale has not yet been proven. Mongin et al. (2021) for example claimed that many fertilizers would sink down before they could be utilized by phytoplankton, and their model study showed that this might reduce potential CO2 uptake by half. There are furthermore questions about the durability of carbon sequestration through the sedimentation of dead biomatter (Fuss et al. 2018). The IPCC AR6 WG3 report assigns it very low technological readiness of 1 to 2 (p116).
Technological Readiness Level (TRL)
A technology with a TRL of 1-3: TRL 1 – Basic; TRL 2 – Concept formulated; TRL 3 – Experimental proof of concept

Scalability
Unknown 0

Timeliness for near-future effects
Unknown 0

Northern + Arctic potential
Unknown 0

Global potential
Unknown 0
In their 2008 report, the Royal Society estimates that by 2100, ocean fertilization would be able to sequester up to 3.7 GtCO2 per year (Lampitt et al, 2008). The IPCC AR6 WG3 report notes that experimental results show a far lower efficiency than theoretical calculations, and ultimately estimates carbon uptake potential of 1 to 3 Gt CO2 per year. However the GESAMP report on marine geoengineering technologies states that 'degree of enhancement of the biological pump varied considerably between experiments', with findings anywhere between a 50 and 8 percent enhancement (GESAMP, 2019). It furthermore also needs to be clarified how much carbon could be released back into the atmosphere when biomass breaks down and the captured carbon is respirated back to higher oceanic layers, and if increased bioproductivity causes a greater emission of other GHGs like methane (GESAMP, 2019).

Cost - benefit
Unknown 0

Environmental risks
Medium 2
The oceans remain largely understudied, and tinkering with lower entropic levels could have major consequences for the entire system and might seriously impact local ecosystems (Boyd et al, 2022; IPCC AR6 WG3, 2022). Fertilization might for example cause environmental damage by causing toxic algae blooms (Wallace et al, 2010; Bertram et al., 2010) and ocean acidification (Williamson and Turley, 2012). The NASEM report thereby attributes a medium level of environmental risk to this measure (2021).
Environmental risks
More widespread and possibly regional impacts that extend beyond the immediate solution deployment location

Community impacts
Unknown 0

Ease of reversibility
Unknown 0

Risk of termination shock
Unknown 0

Legality/governance
Unknown 0

Scientific/media attention
High 3
Scientific/media attention
Numerous scientific papers with substantial funding and ongoing research groups; significant media attention and "hype"; many companies exploring commercialization options
References
https://www.geoengineeringmonitor.org/wp-content/uploads/2021/04/ocean-fertilization.pdf
Batres, M., Wang, F. M., Buck, H., Kapila, R., Kosar, U., Licker, R., ... & Suarez, V. (2021). Environmental and climate justice and technological carbon removal. The Electricity Journal, 34(7), 107002. https://doi.org/10.1016/j.tej.2021.107002
Bertram, C. (2010). Ocean iron fertilization in the context of the Kyoto protocol and the post-Kyoto process. Energy Policy, 38(2), 1130-1139. https://doi.org/10.1016/j.enpol.2009.10.065
Boyd, P. W., Jickells, T., Law, C. S., Blain, S., Boyle, E. A., Buesseler, K. O., ... & Watson, A. J. (2007). Mesoscale iron enrichment experiments 1993-2005: synthesis and future directions. science, 315(5812), 612-617. http://doi.org/10.1126/science.1131669
Cooley, S. R., Klinsky, S., Morrow, D. R., & Satterfield, T. (2023). Sociotechnical considerations about ocean carbon dioxide removal. Annual Review of Marine Science, 15, 41-66. http://doi.org/10.1146/annurev-marine-032122-113850
Emerson D (2019) Biogenic Iron Dust: A Novel Approach to Ocean Iron Fertilization as a Means of Large Scale Removal of Carbon Dioxide From the Atmosphere. Front. Mar. Sci. 6:22. https://doi.org/10.3389/fmars.2019.00022
Fuss, S., Lamb, W. F., Callaghan, M. W., Hilaire, J., Creutzig, F., Amann, T., ... & Minx, J. C. (2018). Negative emissions—Part 2: Costs, potentials and side effects. Environmental Research Letters, 13(6), 063002. https://doi.org/10.1088/1748-9326/aabf9f
GESAMP (2019). “High level review of a wide range of proposed marine geoengineering techniques”. (Boyd, P.W. and Vivian, C.M.G., eds.). (IMO/FAO/UNESCO-IOC/UNIDO/WMO/IAEA/UN/UN Environment/ UNDP/ISA Joint Group of Experts on the Scientific Aspects of Marine Environmental Protection). Rep. Stud. GESAMP No. 98, 144 p. Available at: http://www.gesamp.org/publications/high-level-review-of-a-wide-range-of-proposed-marine-geoengineering-techniques [Accessed 18 July 2024]
Ito, A., Ye, Y., Baldo, C. et al. Ocean fertilization by pyrogenic aerosol iron. npj Clim Atmos Sci 4, 30 (2021). https://doi.org/10.1038/s41612-021-00185-8///Boyd, P.W., Bach, L.T., Hurd, C.L. et al. Potential negative effects of ocean afforestation on offshore ecosystems. Nat Ecol Evol 6, 675–683 (2022). https://doi.org/10.1038/s41559-022-01722-1
Lampitt, R. S., Achterberg, E. P., Anderson, T. R., Hughes, J. A., Iglesias-Rodriguez, M. D., Kelly-Gerreyn, B. A., ... & Yool, A. (2008). Ocean fertilization: a potential means of geoengineering?. Philosophical Transactions of the Royal Society A: Mathematical, Physical and Engineering Sciences, 366(1882), 3919-3945. https://doi.org/10.1098/rsta.2008.0139
Mongin, M., Baird, M. E., Lenton, A., Neill, C., & Akl, J. (2021). Reversing ocean acidification along the Great Barrier Reef using alkalinity injection. Environmental Research Letters, 16(6), 064068. https://doi.org/10.1088/1748-9326/ac002d
Oschlies, A., Koeve, W., Rickels, W., & Rehdanz, K. (2010). Side effects and accounting aspects of hypothetical large-scale Southern Ocean iron fertilization. Biogeosciences, 7(12), 4017-4035. https://doi.org/10.5194/bg-7-4017-2010
Readfearn, Graham (23 December 2021). Can fake whale poo experiment net Australian scientists a share of Elon Musk’s US$100m climate prize? The Guardian https://www.theguardian.com/environment/2021/dec/24/can-fake-whale-poo-experiment-net-australian-scientists-a-share-of-elon-musks-us100m-climate-prize
Silverman-Roati, K., Webb, R. M., & Gerrard, M. (2022). Removing Carbon Dioxide Through Ocean Fertilization: Legal Challenges and Opportunities. Available at: https://scholarship.law.columbia.edu/faculty_scholarship/3637
Smetacek, V., Klaas, C., Strass, V. H., Assmy, P., Montresor, M., Cisewski, B., ... & Wolf-Gladrow, D. (2012). Deep carbon export from a Southern Ocean iron-fertilized diatom bloom. Nature, 487(7407), 313-319. https://doi.org/10.1038/nature11229
Tagliabue, A. et al. 2017. The integral role of iron in ocean biogeochemistry. Nature 543, 51–59. https://doi.org/10.1038/nature21058
DWR Wallace, CS Law, PW Boyd, Y Collos, P Croot, K Denman, PJ Lam, U Riebesell, S Takeda, & P Williamson: 2010. Ocean Fertilization. A Scientific Summary for Policy Makers. IOC/UNESCO, Paris (IOC/BRO/2010/2). Available at: https://unesdoc.unesco.org/ark:/48223/pf0000190674 [Accessed 18 July 2024]
Williamson, P., & Turley, C. (2012). Ocean acidification in a geoengineering context. Philosophical Transactions of the Royal Society A: Mathematical, Physical and Engineering Sciences, 370(1974), 4317-4342. https://doi.org/10.1098/rsta.2012.0167