Space-based solar radiation management
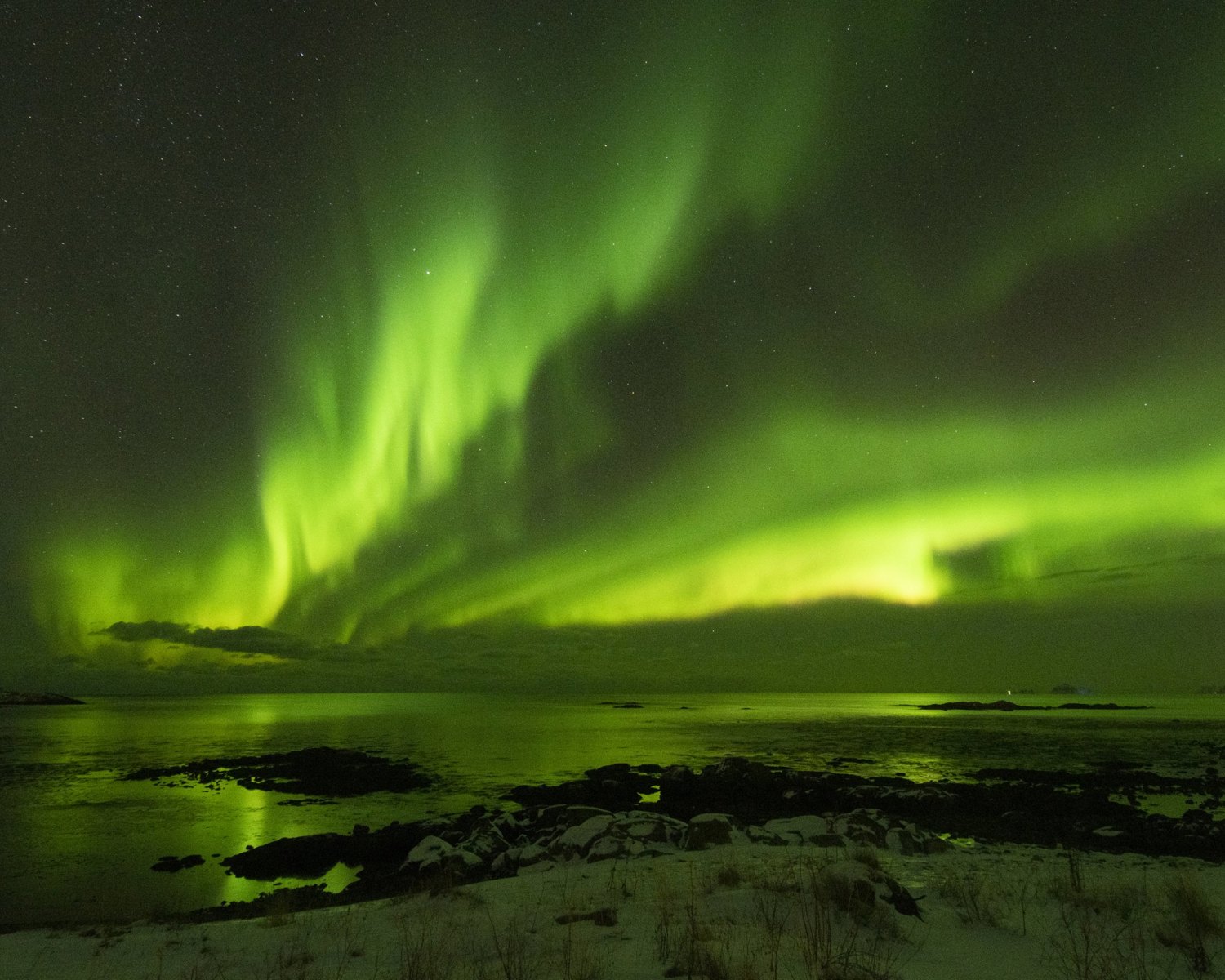
One of the most intuitive SRM approaches would be to reflect or block some solar energy before it reaches the Earth’s atmosphere.
Several space-based ideas have been suggested to do just that (See Baum et al. 2022 for a summary of all ideas). Most of these intend to place something between the Earth and the sun at Lagrange Point L1. These could be space mirrors (Early 1989), Lightsails (Kennedy et al. 2013), space bubbles (https://senseable.mit.edu/space-bubbles/), sunshades (Angel 2006), a fleet of self-reproducing space vehicles (Ellery 2016), or lunar dust (Bromley et al. 2023). Almost all studies share the aim to reduce solar radiation by 1.8%, and claim this would be needed to compensate for a doubling of CO2. Such space-based SRM could have certain advantages over other kinds of SRM, as they would lead to less side effects and be more predictable and they could perhaps be focused on specific areas (Keith 2000).
Analysis overview

Technological Readiness Level (TRL)
Low 1
Some of the earliest space based geoengineering ideas were developed during the early 20th century, and were given an impulse by the Space Race, with several wild speculative projects being suggested in the USSR (see Keith, 2000; Fleming, 2010). These past and present ideas are however largely standalone explorations that are very far from development. A 2021 report by RAND estimated the technological readiness of space mirrors as medium, but it is unclear why they think so other than that some smaller space mirrors already exist. Bromley et al’s (2023) comments are illustrative of the overestimations around the feasibility of such projects as they write that ‘[r]oughly 10 [to the power of ] 10 kg of dust per year is needed for Earth-climate impact, which is approximately 700 times more mass than humans have launched into space', and that the 'easier' alternative therefore would be to use mined lunar dust and launch it ‘on ballistic trajectories that cross near the Earth-Sun line of sight.'
In general most reviewers dismiss such techniques as an option. UNEP (2023) observes that the developmental timescales ‘appear prohibitive compared to other approaches’, and NASEM (2015) chose not to consider space-based ideas ‘because of the substantial time (>20 years) … and technology challenges associated with these issues’. Baum et al, (2022) analysis of expert opinions found that although many were ‘broadly positive about the concept itself’, they were ‘unsure about its ultimate workability.'
Technological Readiness Level (TRL)
A technology with a TRL of 1-3: TRL 1 – Basic; TRL 2 – Concept formulated; TRL 3 – Experimental proof of concept

Scalability
Medium 2
Scalability
Physically somewhat scalable; linear efficiency

Timeliness for near-future effects
Low 1
Timeliness for near-future effects
Implemented too late to make a significant difference

Northern + Arctic potential
High 3
Northern + Arctic potential
Very detectable impacts in the Arctic, above the global average; technology ideally/preferably located here

Global potential
High 3
Global potential
Major impacts detected

Cost - benefit
Prohibitive 1
Cost - benefit
Cost of investment comparable to cost of avoided damage

Environmental risks
Low risk 3
Environmental risks
Very limited, site-specific effects restricted to the solution deployment location only

Community impacts
Unknown 0

Ease of reversibility
Unknown 0

Risk of termination shock
High risk 1
Risk of termination shock
High or very significant termination shock or damage

Legality/governance
Unknown 0

Scientific/media attention
Medium 2
Baum et al (2022) notes that 'the literature on space-based geoengineering is limited', and that only 2% of articles on geoengineering consider space-based methods. Some spectacular ideas like Bromley et al’s (2023) space dust from the moon idea have reached the public media, but as Keith et al (2020) write: ‘For the most part [such ideas] are simply left unconsidered.’
Scientific/media attention
Some attention within the scientific community, including published research and funding programmes; some media attention; some commercial interest
References
Angel, R. (2006). Feasibility of cooling the Earth with a cloud of small spacecraft near the inner Lagrange point (L1). Proceedings of the National Academy of Sciences, 103(46), pp.17184-17189. https://doi.org/10.1073/pnas.0608163103
Baum, C. M., Low, S., & Sovacool, B. K. (2022). Between the sun and us: Expert perceptions on the innovation, policy, and deep uncertainties of space-based solar geoengineering. Renewable and Sustainable Energy Reviews, 158, 112179. https://doi.org/10.1016/j.rser.2022.112179
Bromley BC, Khan SH, Kenyon SJ (2023) Dust as a solar shield. PLOS Clim 2(2): e0000133. https://doi.org/10.1371/journal.pclm.0000133
Ellery, A., 2016. Low-Cost Space-Based Geoengineering: An assessment based on self-replicating manufacturing of in-situ resources on the moon. World Academy of Science, Engineering and Technology International Journal of Environmental and Ecological Engineering, 10(2), pp.278-285. https://doi.org/10.1017/S1473550422000234
Fleming, J. R. (2010). Fixing the sky: the checkered history of weather and climate control. Columbia University Press.
Grisé, Michelle, Emmi Yonekura, Jonathan S. Blake, David DeSmet, Anusree Garg, and Benjamin Lee Preston, Climate Control: International Legal Mechanisms for Managing the Geopolitical Risks of Geoengineering. Santa Monica, CA: RAND Corporation, 2021. https://www.rand.org/pubs/perspectives/PEA1133-1.html.
Keith, D. W. (2000). Geoengineering the climate: History and prospect. Annual review of energy and the environment, 25(1), 245-284. https://doi.org/10.1146/annurev.energy.25.1.245
Keith, D. W., Morton, O., Shyur, Y., Worden, P., & Wordsworth, R. (2020, March 17). Reflections on a meeting about space-based solar geoengineering. Harvard’s Solar Geoengineering Research Program. https://geoengineering.environment.harvard.edu/blog/reflections-meeting-about-space-based-solargeoengineering
Kennedy III, R. G., Roy, K. I., & Fields, D. E. (2013). Dyson Dots: Changing the solar constant to a variable with photovoltaic lightsails. Acta Astronautica, 82(2), 225-237. https://doi.org/10.1016/j.actaastro.2012.10.022
National Academies of Sciences, Engineering, and Medicine. 2015. Climate Intervention: Reflecting Sunlight to Cool Earth. Washington, DC: The National Academies Press. https://doi.org/10.17226/18988
Yonekura, Emmi. (2022). Why Not Space Mirrors? The RAND Blog, October 19. Available at: https://www.rand.org/blog/2022/10/why-not-space-mirrors.html