Bio-energy with carbon storage BECCS
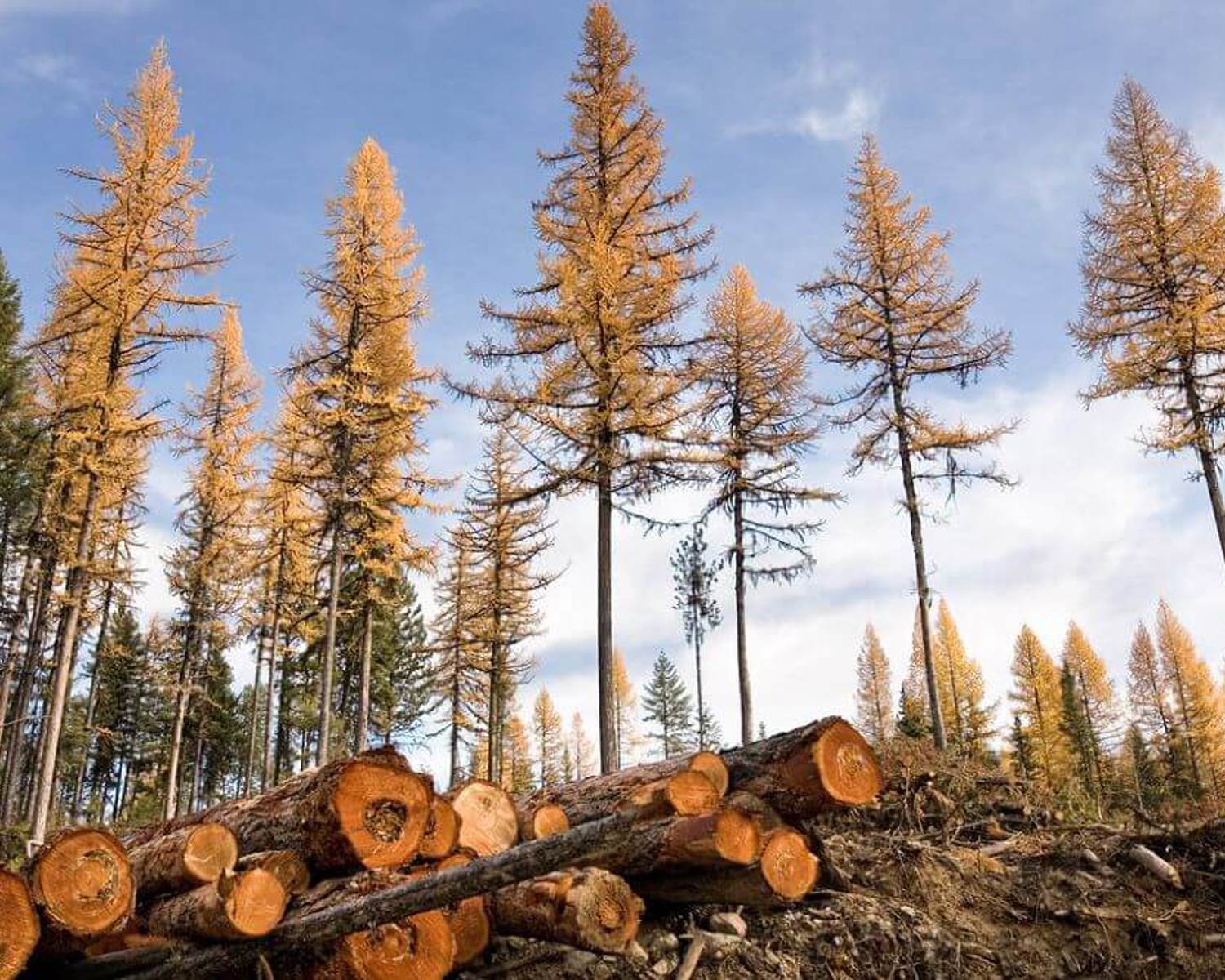
Sustainable forest management involves the maintenance and enhancement of forest environments, ensuring longevity of forest ecosystems while allowing the best possible environmental, economic, social and cultural opportunities now and into the future. In Canada, the world’s largest exporter of forest products, harvest rates and strict jurisdictions are set to ensure long term ecosystem sustainability, protecting soil and water resources and 55% of all energy used by the forestry sector is renewable bio-energy.
Year: 2014
Photographer: Lawrence Hislop
Bio-Energy with Carbon Storage (BECCS) offers a nature-based way to remove CO2 from the atmosphere by consuming biomaterial and removing the remaining carbon residues from the carbon cycle.
The biomass can be turned into energy through various ways, such as burning it, gasification, or fermentation. The CO2 released from consumption of the biomass would preferably be captured to a large degree if the net positive effects of the biomass capture would not be negated. This could be done through similar methods already in use for Carbon Capture and Storage by the fossil fuel industry.
Analysis overview

Technological Readiness Level (TRL)
Medium 2
Technological Readiness Level (TRL)
A technology with a TRL of 4-6: TRL 4 – validated in lab; TRL 5 – validated in relevant environment; TRL 6 – demonstrated in relevant environment

Scalability
Medium 2
Although BECCS play an important part in all negative emission scenarios that aim to keep temperature rises within an acceptable level, serious concerns remain around the potential and effect of its scalability (Ekardt and von Bredow, 2012; Vaughan and Gough, 2016; Mander et al. 2017).
A main downside to BECCS is that it would use large volumes of biomass, and would therefore need space and nutrients that could otherwise be used to produce resources, food, or provide other ecosystem services (RoyalSociety 2018). This large-scale deployment of BECCS and the required amounts of land could also have significant negative climate effects (Newbold et al, 2015) and might affect albedo (See Bio-geoengineering to increase crop albedo), especially at high-latitudes (Fuss et al., 2018). Williamson (2016) also warns that biomass might not be as efficient at capturing CO2 at scale for use in BECCS and that the response of plants to future climate change needs to be taken into consideration. The production sites might furthermore be far removed from the places of energy generation, and might therefore come with significant extra transportation costs. These are now estimated to be around 100 to 200/tCO2 (Fuss et al. 2018). Fridahl and Lehtveer (2018), moreover, find important socio-political constraints to BECCS deployment, and highlight the need for further research into factors such as social acceptance or policy development. Chiquier et al. (2022) notes that while ‘BECCS delivers immediate and permanent CDR’, ‘its CO2 removal efficiency can be significantly impacted by any initial carbon debt associated with (direct and indirect) land use change, and thereby significantly delayed’.
Scalability
Physically somewhat scalable; linear efficiency

Timeliness for near-future effects
High 3
There are already several operational plants, but captured amounts remain minimal, and questions about scalability remain (Smith et al. 2023).
Timeliness for near-future effects
Implemented in time to make a significant difference

Northern + Arctic potential
Medium 2
Northern + Arctic potential
Statistically detectable impacts in the Arctic above the global average; no difference between deploying the solution here or elsewhere

Global potential
Medium 2
There is significant uncertainty about the potential of BECCS (Anderson and Peters, 2016; Hanssen et al. 2020), with the IPCC AR6 WG3 (2022) following the estimates of the State of Carbon Dioxide Removal report of a carbon capture potential of 0.5 to 11 GtCO2/yr (Smith et al. 2023). There are many variables that would decide the effectiveness of BECCS, with Vaughan et al’s (2018) model study for instance showing poor governance can significantly reduce the CO2 capture potential.
Global potential
Statistically detectable impacts

Cost - benefit
Medium 2
The State of Carbon Dioxide Removal report estimates potential costs of 15 to 400 $/tCO2 (Smith et al. 2023). Yet, as with biochar application (See biochar), significant uncertainties remain about the costs of BECCS deployment as there are major differences between individual projects, and much research is still needed into costs, and designing strategies that could pay for BECCS (Honegger et al. 2021). Möllersten and Naqvi reported in an overview study (2022) that estimated costs of BECCS deployment in the Nordic region would be around 60-135 USD/tCO2.
Cost - benefit
Significant investment costs needed, but still much cheaper than the avoided damage costs (e.g., 30%).

Environmental risks
Medium 2
The environmental effects of large scale BECCS deployment could be significant as unsustainable and large scale biomass harvesting could cause competition for land and water and reduce biodiversity and soil fertility (Smith et al, 2023 IPCC AR6,WG3 2022). Crucially, specific parts of the BECCS chain could be more or less sustainable, and it has to be made sure that the process as a whole has positive effects (Fajardy and Dowell, 2017; Briones-Hidrovo et al. 2022). Briones-Hidrovo et al. (2022) for example note that the use of residual forest biomass had a positive climate impact, but also had significant impact on land and water. The use of contaminated biomass could moreover incur pollution risks (Smith et al. 2023), and Deng et al (2017) point to significant risks of leakage if captured carbon needs to be transported.
Environmental risks
More widespread and possibly regional impacts that extend beyond the immediate solution deployment location

Community impacts
Unknown 0
BECCS could have positive and negative effects on local communities.Small scale bioenergy development could provide extra local income (Almena et al,.2022). And a sound growth process could improve crop growth and health, enhance biodiversity, soil health, and water quality (Smith et al, 2023). However, as large-scale BECCS deployment would require significant amounts of land and water Günther and Ekardt (2022) point out that it can have significant detrimental impacts for communities, and even violate their basic human rights like the rights to food, water, and a healthy environment. The IPCC AR6 (2022) report therefore warns that poorly implemented biomass growth for BECCS could have significant negative effects on ‘local livelihoods and on the rights of Indigenous Peoples, especially if implemented at large scales and where land tenure is insecure (high confidence).'

Ease of reversibility
Medium 2

Risk of termination shock
Low 3
Risk of termination shock
Low or insignificant termination shock or damage

Legality/governance
High 3
Legality/governance
Currently legal to deploy, with governance structures in place to facilitate it and/or financial incentives to develop it

Scientific/media attention
High 3
Scientific/media attention
Numerous scientific papers with substantial funding and ongoing research groups; significant media attention and "hype"; many companies exploring commercialization options
References
Almena, A., Thornley, P., Chong, K., & Röder, M. (2022). Carbon dioxide removal potential from decentralised bioenergy with carbon capture and storage (BECCS) and the relevance of operational choices. Biomass and Bioenergy, 159, 106406. https://doi.org/10.1016/j.biombioe.2022.106406
Anderson, K., & Peters, G. (2016). The trouble with negative emissions. Science, 354(6309), 182-183. https://doi.org/10.1126/science.aah456
C. Azar, K. Lindgren, E. Larson, K. Möllersten Carbon capture and storage from fossil fuels and biomass – costs and potential role in stabilizing the atmosphere Climatic Change, 74 (1) (2006), pp. 47-79. https://link.springer.com/article/10.1007/s10584-005-3484-7
Briones-Hidrovo, A., Rey, J. R. C., Dias, A. C., Tarelho, L. A., & Beauchet, S. (2022). Assessing a bio-energy system with carbon capture and storage (BECCS) through dynamic life cycle assessment and land-water-energy nexus. Energy Conversion and Management, 268, 116014. https://doi.org/10.1016/j.enconman.2022.116014
Chiquier, S., Patrizio, P., Bui, M., Sunny, N., & Mac Dowell, N. (2022). A comparative analysis of the efficiency, timing, and permanence of CO 2 removal pathways. Energy & Environmental Science, 15(10), 4389-4403. https://doi.org/10.1039/D2EE01021F
Deng, H.; Bielicki, J.M.; Oppenheimer, M.; Fitts, J.P.; Peters, C.A. Leakage Risks of Geologic CO2 Storage and the Impacts on the Global Energy System and Climate Change Mitigation. Clim. Change 2017, 144, 151–163. https://doi.org/10.1007/s10584-017-2035-8
Ekardt, F.; von Bredow, H. Extended Emissions Trading Versus Sustainability Criteria: Managing the Ecological and Social Ambivalence of Bioenergy. Renew. Energy Law Policy Rev. 2012, 3, 49–64. Available at: https://www.researchgate.net/publication/281644028_Extended_emissions_trading_versus_sustainability_criteria_Managing_the_ecological_and_social_ambiva-_lences_of_bioenergy [Accessed 22 July 2024]
Fajardy, M., & Mac Dowell, N. (2017). Can BECCS deliver sustainable and resource efficient negative emissions?. Energy & Environmental Science, 10(6), 1389-1426. https://doi.org/10.1039/C7EE00465F
Fridahl, M., & Lehtveer, M. (2018). Bioenergy with carbon capture and storage (BECCS): Global potential, investment preferences, and deployment barriers. Energy Research & Social Science, 42, 155-165. https://doi.org/10.1016/j.erss.2018.03.019
Fuss, S.; Lamb, W.F.; Callaghan, M.W.; Hilaire, J.; Creutzig, F.; Amann, T.; Beringer, T.; De Oliveira Garcia, W.; Hartmann, J.; Khanna, T.; et al. Negative Emissions—Part 2: Costs, Potentials and Side Effects. Environ. Res. Lett. 2018, 13, 063002. https://doi.org/10.1088/1748-9326/aabf9f
Günther, P., & Ekardt, F. (2022). Human Rights and Large-Scale Carbon Dioxide Removal: Potential Limits to BECCS and DACCS Deployment. Land, 11(12), 2153. https://doi.org/10.3390/land11122153
Hanssen, S. V., Daioglou, V., Steinmann, Z. J. N., Doelman, J. C., Van Vuuren, D. P., & Huijbregts, M. A. J. (2020). The climate change mitigation potential of bioenergy with carbon capture and storage. Nature Climate Change, 10(11), 1023-1029. https://doi.org/10.1038/s41558-020-0885-y
Honegger, M.; Poralla, M.; Michaelowa, A.; Ahonen, H.M. Who Is Paying for Carbon Dioxide Removal? Designing Policy Instruments for Mobilizing Negative Emissions Technologies. Front. Clim. 2021, 3, 672996. https://doi.org/10.3389/fclim.2021.672996
Intergovernmental Panel on Climate Change (IPCC). Climate Change 2022. Mitigation of Climate Change. Working Group III Contribution to the Sixth Assessment Report of the Intergovernmental Panel on Climate Change; Intergovernmental Panel on Climate Change (IPCC): Geneva, Switzerland, 2022. Available at: https://www.ipcc.ch/report/sixth-assessment-report-working-group-3/ [Accessed 22 July 2024]
Newbold, T., Hudson, L. N., Hill, S. L., Contu, S., Lysenko, I., Senior, R. A., ... & Purvis, A. (2015). Global effects of land use on local terrestrial biodiversity. Nature, 520(7545), 45-50. https://doi.org/10.1038/nature14324
Pires, J. C. M. (2019). Negative emissions technologies: a complementary solution for climate change mitigation. Science of the Total Environment, 672, 502-514. https://doi.org/10.1016/j.scitotenv.2019.04.004
Pruess, K.; García, J. Multiphase Flow Dynamics during CO2 Disposal into Saline Aquifers. Environ. Geol. 2002, 42, 282–295. https://doi.org/10.1007/s00254-001-0498-3
Smith, S. M., Geden, O., Nemet, G., Gidden, M., Lamb, W. F., Powis, C., Bellamy, R., Callaghan, M., Cowie, A., Cox, E., Fuss, S., Gasser, T., Grassi, G., Greene, J., Lück, S., Mohan, A., Müller-Hansen, F., Peters, G., Pratama, Y., Repke, T., Riahi, K., Schenuit, F., Steinhauser, J., Strefler, J., Valenzuela, J. M., and Minx, J. C. (2023). The State of Carbon Dioxide Removal - 1st Edition. The State of Carbon Dioxide Removal. https://doi.org/10.17605/OSF.IO/W3B4Z
Vaughan, N. E., & Gough, C. (2016). Expert assessment concludes negative emissions scenarios may not deliver. Environmental research letters, 11(9), 095003. https://doi.org/10.1088/1748-9326/11/9/095003
Vaughan, N. E., Gough, C., Mander, S., Littleton, E. W., Welfle, A., Gernaat, D. E., & Van Vuuren, D. P. (2018). Evaluating the use of biomass energy with carbon capture and storage in low emission scenarios. Environmental Research Letters, 13(4), 044014. https://doi.org/10.1088/1748-9326/aaaa02
Vogt, R. D., de Wit, H., & Koponen, K. (2022). Case study on impacts of large-scale re-/afforestation on ecosystem services in Nordic regions. NEGEM-Quantifying and Deploying Responsible Negative Emissions in Climate Resilient Pathways. Horizon2020, Grant Agreement no. 869192. Available at: https://www.negemproject.eu/wp-content/uploads/2023/05/D-3.6-Case-study-on-impacts-of-large-scale-reforestation-afforestation-in-Nordic-Countries.pdf [Accessed 22 July 2024]
Williamson, P. (2016). Emissions reduction: scrutinize CO2 removal methods. Nature, 530(7589), 153-155. https://doi.org/10.1038/530153a