Enhancing permafrost refreezing with air pipes
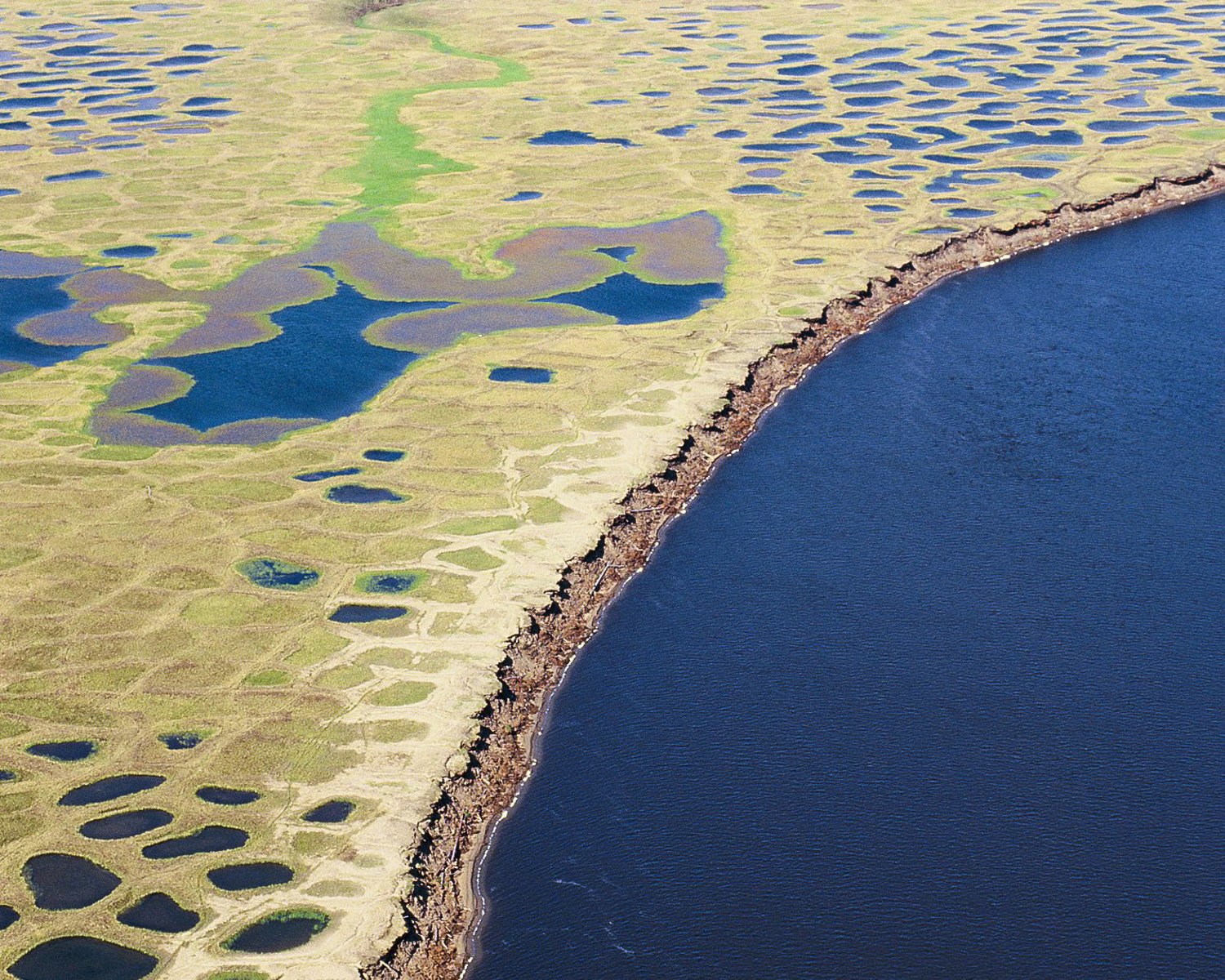
Thermosyphon technologies that passively cool soils if the air temperature is colder than surface temperatures have been used on a smaller scale to stabilize permafrost that supports infrastructure (Xu and Goering, 2008).
The largest example of thermosyphon usage is the Trans Alaska Pipeline which uses such systems along its 1300 km track. There have been some isolated online suggestions to use such a technology on a larger scale to stabilize Northern permafrost. Similarly, there has been a suggestion to use passive air cooling with large ceramic half-pipes built into the permafrost (https://klinkmansolar.com/kfrozen.htm).
Analysis overview

Technological Readiness Level (TRL)
Low 1
Technological Readiness Level (TRL)
A technology with a TRL of 1-3: TRL 1 – Basic; TRL 2 – Concept formulated; TRL 3 – Experimental proof of concept

Scalability
Low 1
Scalability
Physically unable to scale; sub-linear/logarithmic efficiency of scalability

Timeliness for near-future effects
Low 1
Timeliness for near-future effects
Implemented too late to make a significant difference

Northern + Arctic potential
Low 1
Northern + Arctic potential
No noticeable extra positive effect beyond the global average; technology is unsuited to the Arctic

Global potential
Low 1

Cost - benefit
Prohibitive 1
Cost - benefit
Cost of investment comparable to cost of avoided damage

Environmental risks
Unknown 0

Community impacts
Unknown 0

Ease of reversibility
Hard 1
Ease of reversibility
Impossible or very difficult to reverse

Risk of termination shock
Low risk 3
Risk of termination shock
Low or insignificant termination shock or damage

Legality/governance
Possible 3
Legality/governance
Currently legal to deploy, with governance structures in place to facilitate it and/or financial incentives to develop it

Scientific/media attention
Low 1
Scientific/media attention
Very low attention from individuals and/or abandoned ideas; low media attention; no commercial interest.
References
Wagner, A. M., Maakestad, J. B., Yarmak, E., & Douglas, T. A. (2021). Artificial ground freezing using solar-powered thermosyphons. US Army Corps of Engineers. Washington, DC. Available at: https://apps.dtic.mil/sti/trecms/pdf/AD1153377.pdf [Accessed 19 July 2024]
Xu, J., & Goering, D. J. (2008). Experimental validation of passive permafrost cooling systems. Cold Regions Science and Technology, 53(3), 283-297. https://doi.org/10.1016/j.coldregions.2007.09.002
Zueter, A. F., & Sasmito, A. P. (2023). Cold energy storage as a solution for year-round renewable artificial ground freezing: Case study of the Giant Mine Remediation Project. Renewable Energy, 203, 664-676. https://doi.org/10.1016/j.renene.2022.12.093