Agricultural soil management
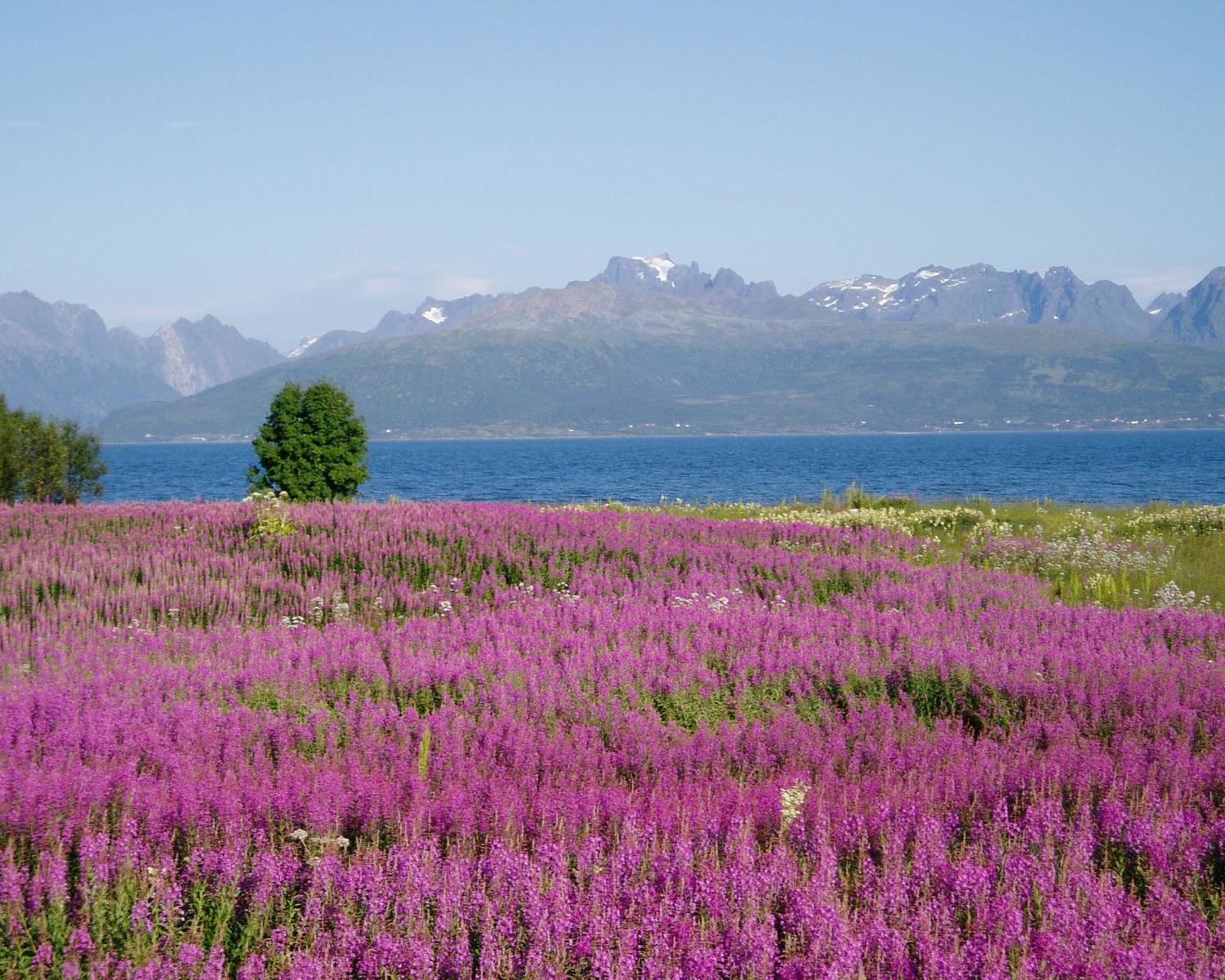
Terrestrial carbon can be stored in biomass above or below the ground, and in soils themselves. Soil organic matter can form differently, and have different amounts of plant and microbial components depending on the availability of water (Cotrufo and Lavallee, 2022). The large amounts of the Earth that have been brought under cultivation over the past 12.000 years have significantly degraded soil carbon levels, and have released some 110 billion metric tons of carbon (Sanderman et al. 2017). Soil security and health is increasingly being recognised as essential for planetary health (Kopittke et al. (2022).
Agricultural soil management, as considered here, seeks to increase soil carbon content, and thereby reduce atmospheric carbon levels. There are various different methods to do so, like shifts in fertilization practices, soil tillage practices, and crop management practices, with specific strategies depending on local conditions and desired outcomes (Lessmann et al. 2022). There are therefore many links to other land management practises (see, for example, Afforestation and Conservation and restoration of peatlands restoration), as well as albedo enhancing strategies like the use of cover crops to influence albedo (Lugato et al. 2020; see Bio-geoengineering to increase crop albedo) and sometimes biochar application is also considered under this category (see Biochar). Amelung et al. (2020) note that such soil management can be especially effective in cropland soils ‘with large yield gaps and/or large historic soil organic carbon losses’.
Analysis overview

Technological Readiness Level (TRL)
High 3
Straightforward soil management practices are already very well developed, and the IPCC AR6 WG3 therefore assigns a TRL of 8/9 to Soil carbon sequestration in croplands and grasslands. Paustian et al (2019) however notes that some to-be-developed technologies might be even more effective.
Technological Readiness Level (TRL)
A technology with a TRL of 7-9: TRL 7 – prototype demonstrated; TRL 8 – system complete; TRL 9 – system proven

Scalability
Medium 2
IPCC AR6 WG3 notes that ‘Regionally, soil carbon management in croplands and grasslands is feasible anywhere, but effectiveness can be limited in very dry regions, and for grasslands it is greatest in areas where degradation has occurred (e.g., by overgrazing) and soil organic carbon is depleted.’
Scalability
Physically somewhat scalable; linear efficiency

Timeliness for near-future effects
High 3
IPCC AR6 WG3 has medium to high confidence that this measure could deliver 0.6–9.3 (GtCO2 yr–1). Paustian et al. (2019) literature review states that ‘There is a strong scientific basis for managing agricultural soils to act as a significant carbon (C) sink over the next several decades.’ Beyond this, more novel technologies might enhance effectiveness even further.
Timeliness for near-future effects
Implemented in time to make a significant difference

Northern + Arctic potential
Low 1
Because the extent of cropland is limited in the Northern and Arctic region, the effects will likely be less there than in other areas of the globe. However, many foresee a northward expansion of agriculture under warming climate conditions (Unc et al (2021); Meyfroidt, 2021; Bradley and Stein, 2022; Angers et al, 2022). Unc et al (2021) notes that although this might provide food for 0.25 to 1 billion people, it also risks releasing up to 76% of vegetation and soil carbon there by 2100. This would make soil carbon management practices more relevant for the region.However, much more research would be needed, as at present, there are already large uncertainties around general soil carbon losses in the Arctic due to climate warming (Wieder et al, 2019).
Northern + Arctic potential
No noticeable extra positive effect beyond the global average; technology is unsuited to the Arctic

Global potential
Medium 2
The IPCC AR6 WG3 gives a broad range for the global potential of Soil carbon sequestration in croplands and grasslands of 0.6 to 9.3 (GtCO2 yr–1). Fuss et al. (2018) carbon mitigation overview study estimates 0.4 to 0.8 Gt C yr−1. Lessmann et al (2022) gives an optimal global potential of 0.44 to 0.68 Gt C yr−1, but cautions that this figure is more realistically 0.28 to 0.43 Gt C yr−1. Beyond this, Paustian et al (2019) literature review notes that the developments of newer technologies might almost double expected carbon mitigation potential in a couple of decades.
Guenet et al. (2021) caution that this should be done without increasing fertilizer inputs, as related N2O emissions might offset carbon mitigation gains. Related to this, Leifeld and Menichetti (2018) show that peatland restoration would have a similar sequestration potential, but would require far less land and 3.4 times less nitrogen.
Global potential
Statistically detectable impacts

Cost - benefit
Cost-effective 3
The IPCC AR6 WG3 estimates a cost of negative 45 to plus 100 (USD tCO2–1), depending on the local specificities and potential economic side benefits. Tang et al (2016) give estimates in the range of 3 to 130 USD tCO2–1.
Cost - benefit
Low investment cost compared to the avoided damage cost (e.g., a few %) and/or inexpensive relative to other measures with similar impact

Environmental risks
Low risk 3
Environmental risks
Very limited, site-specific effects restricted to the solution deployment location only

Community impacts
Beneficial 3
Community impacts
Significant benefits to communities

Ease of reversibility
Easy 3

Risk of termination shock
Low risk 3
Risk of termination shock
Low or insignificant termination shock or damage

Legality/governance
Possible 3
Unc et al. (2021) write that there are significant differences between countries. As ‘Finland, Sweden, and Denmark support agricultural intensification through the development of resilient farming systems and food value chains that consider changing dietary preferences and impact of land use and land use changes on biogeochemical cycles’, while ‘in the Canadian prairies and Mongolia, legislation favours the northward areal expansion of commercial agriculture, even in the absence of explicit policies or strong local population pressures.’
Legality/governance
Currently legal to deploy, with governance structures in place to facilitate it and/or financial incentives to develop it

Scientific/media attention
High 3
Apart from broad scientific interest, there is increasing attention for soil management by the public and politicians. This is partially due to large international programs like the UNFCC’ 4p1000 initiative (https://4p1000.org/), and popular grassroots campaigns like the one started by the popular figure Sadguru (https://consciousplanet.org).
Scientific/media attention
Numerous scientific papers with substantial funding and ongoing research groups; significant media attention and "hype"; many companies exploring commercialization options
References
Amelung, W., Bossio, D., de Vries, W., Kögel-Knabner, I., Lehmann, J., Amundson, R., ... & Chabbi, A. (2020). Towards a global-scale soil climate mitigation strategy. Nature communications, 11(1), 5427. https://doi.org/10.1038/s41467-020-18887-7
Angers, D., Ouimet, R., Roy-Léveillée, P., & Garneau, M. (2022). Priorities for management and protection of Québec soils. Geoderma Regional, 29, e00523. https://doi.org/10.1016/j.geodrs.2022.e00523
Bradley, H., & Stein, S. (2022). Climate opportunism and values of change on the Arctic agricultural frontier. Economic Anthropology, 9(2), 207-222. https://doi.org/10.1002/sea2.12251
Branca, G., Lipper, L., McCarthy, N., & Jolejole, M. C. (2013). Food security, climate change, and sustainable land management. A review. Agronomy for sustainable development, 33, 635-650. https://doi.org/10.1007/s13593-013-0133-1
Cotrufo, M. F., & Lavallee, J. M. (2022). Soil organic matter formation, persistence, and functioning: A synthesis of current understanding to inform its conservation and regeneration. Advances in agronomy, 172, 1-66. https://doi.org/10.1016/bs.agron.2021.11.002
Duarte-Guardia, S., Peri, P., Amelung, W., Thomas, E., Borchard, N., Baldi, G., ... & Ladd, B. (2020). Biophysical and socioeconomic factors influencing soil carbon stocks: a global assessment. Mitigation and Adaptation Strategies for Global Change, 25, 1129-1148. https://doi.org/10.1007/s11027-020-09926-1
Griscom, B. W., Adams, J., Ellis, P. W., Houghton, R. A., Lomax, G., Miteva, D. A., ... & Fargione, J. (2017). Natural climate solutions. Proceedings of the National Academy of Sciences, 114(44), 11645-11650. https://doi.org/10.1073/pnas.1710465114
IPCC (2019): Climate Change and Land: an IPCC special report on climate change, desertification, land degradation, sustainable land management, food security, and greenhouse gas fluxes in terrestrial ecosystems (eds Shukla, P. R. et al.) https://www.ipcc.ch/site/assets/uploads/2019/11/SRCCL-Full-Report-Compiled-191128.pdf.
Kopittke, P. M., Berhe, A. A., Carrillo, Y., Cavagnaro, T. R., Chen, D., Chen, Q. L., ... & Minasny, B. (2022). Ensuring planetary survival: the centrality of organic carbon in balancing the multifunctional nature of soils. Critical Reviews in Environmental Science and Technology, 52(23), 4308-4324. https://doi.org/10.1080/10643389.2021.2024484
Lehmann, J., Cowie, A., Masiello, C. A., Kammann, C., Woolf, D., Amonette, J. E., ... & Whitman, T. (2021). Biochar in climate change mitigation. Nature Geoscience, 14(12), 883-892. https://doi.org/10.1038/s41561-021-00852-8
Leifeld, J., & Menichetti, L. (2018). The underappreciated potential of peatlands in global climate change mitigation strategies. Nature communications, 9(1), 1071. https://doi.org/10.1038/s41467-018-03406-6
Lessmann, M., Ros, G. H., Young, M. D., & de Vries, W. (2022). Global variation in soil carbon sequestration potential through improved cropland management. Global Change Biology, 28(3), 1162-1177. https://doi.org/10.1111/gcb.15954
Meyfroidt, P. (2021). Emerging agricultural expansion in northern regions: Insights from land-use research. One Earth, 4(12), 1661-1664. https://doi.org/10.1016/j.oneear.2021.11.019
Paustian, K., Larson, E., Kent, J., Marx, E., & Swan, A. (2019). Soil C sequestration as a biological negative emission strategy. Frontiers in Climate, 8. https://doi.org/10.3389/fclim.2019.00008
Richards, M., Arslan, A., Cavatassi, R., & Rosenstock, T. (2019). Climate change mitigation potential of agricultural practices supported by IFAD investments. IFAD Res. Ser, 35, 1-30. ISBN 978-92-9072-875-7. Available at: https://www.ifad.org/documents/38714170/41066943/35_research.pdf [Accessed 19 July 2024]
Sanderman, J., Hengl, T., & Fiske, G. J. (2017). Soil carbon debt of 12,000 years of human land use. Proceedings of the National Academy of Sciences, 114(36), 9575-9580. https://doi.org/10.1073/pnas.1706103114
Tang, K., Kragt, M. E., Hailu, A. & Ma, C. 2016. Carbon farming economics: what have we learned? J. Environ. Manag. 172, 49–57. https://doi.org/10.1016/j.jenvman.2016.02.008
Unc, A., Altdorff, D., Abakumov, E., Adl, S., Baldursson, S., Bechtold, M., ... & Borchard, N. (2021). Expansion of agriculture in northern cold-climate regions: a cross-sectoral perspective on opportunities and challenges. Frontiers in Sustainable Food Systems, 5, 663448. https://doi.org/10.3389/fsufs.2021.663448
Wieder, W. R., Sulman, B. N., Hartman, M. D., Koven, C. D., & Bradford, M. A. (2019). Arctic soil governs whether climate change drives global losses or gains in soil carbon. Geophysical Research Letters, 46(24), 14486-14495. https://doi.org/10.1029/2019GL085543